Lack of cardiac benefit after intramyocardial or intravenous injection of mesenchymal stem cell-derived extracellular vesicles supports the need for optimized cardiac delivery
Abstract
Aim: To determine the differences in improvement in cardiac function by intramyocardial (IM) vs. intravenous (IV) injection of human bone mesenchymal stem cell-derived extracellular vesicles (HBMSC-EV) after acute MI.
Methods: FVB mice underwent acute MI via left anterior descending coronary artery ligation and subsequent injection of: (1) IM saline control; (2) IM HBMSC-EV; (3) IV saline control; and (4) IV HBMSC-EV. Cardiac function was evaluated with weekly postoperative echocardiography. On postoperative day 28, the mice were euthanized, and the heart, lungs, liver, spleen, and kidneys were harvested. Given previous studies showing HBMSC-EV hepatic uptake after IV injection, the liver was evaluated for changes in inflammation, fibrosis, and proliferation.
Results: On postoperative day 28, there were no significant differences in left ventricular ejection fraction
Conclusions: Although there was a trend in the improvement in infarct size, a single-dose administration of neither IM nor IV injection of HBMSC-EV resulted in significant improvement in post-MI cardiac function. A major limitation of this study is the lack of trials determining the optimal dose of HBMSC-EV needed in this model. However, the current study demonstrates that future studies are required to either optimize administration or bioengineer HBMSC-EV with cardiac-homing properties.
Keywords
INTRODUCTION
Extracellular vesicles (EV) are secreted by almost every cell type and are lipid bilayer membranes that encase a variety of compounds such as proteins, lipids, nucleic acids, etc. In stem cells, EVs are thought to be responsible for the beneficial paracrine effects. Stem cell-derived EVs have a wide range of therapeutic functions in preclinical models of cardiovascular disease - EVs have been shown to protect several forms of myocardial ischemia in both small and large animal models[1-4].
Furthermore, intramyocardial (IM) injection has been shown to be the most reliable form of EV delivery, while intravenous (IV) injection is not[1,2,4-7]. A systemic review found that when EVs were intravenously injected, there was little to no evidence of EV localization to the heart, with primarily hepatic and splenic uptake[8]. We recently confirmed these findings in a model of murine acute MI, and only found cardiac uptake of EVs after IM injection and not with IV injection[7].
However, no long-term functional studies have been done to compare the effects of intramyocardial vs. IV EV injection side-by-side in an acute MI model. These two different delivery methods are important to compare since IM injection has more reliable cardiac benefits in preclinical studies, but requires a thoracotomy to deliver. IV injection, though with less reliable cardiac benefits, is a non-invasive delivery method that is more translatable to clinical practice. In this study, we delivered human bone mesenchymal stem cell-derived EVs (HBMSC-EV) via either IM or IV injection after murine acute MI and examined cardiac function 28 days post-injection. Additionally, as our previous findings confirmed hepatic HBMSC-EV uptake after IV injection, we evaluated for evidence of changes in liver inflammation, fibrosis, growth, and oxidative status 28 days post-injection since mesenchymal stem cell-derived EVs have been shown to have beneficial effects in preclinical liver disease models[9]. We also wanted to determine if there were any detectable long-term changes in the liver after HBMSC-EV uptake.
METHODS
HBMSC-EV isolation
HBMSC (Lonza, PT-2501) were cultured to passage 7 with the Mesenchymal Stem Cell Growth Medium (MSCGM) BulletKit (Lonza, PT-3001), per manufacturer’s instructions[7]. At 80%-90% confluency, the old MSCGM was removed from the HBMSC and replaced with fresh MSCGM. The HBMSC were placed in a humidified hypoxia chamber (Billups‐Rothenberg, MIC‐101) containing 95% N2 and 5% CO2 for 24 h at
HBMSC-EV characterization
As previously described, the HBMSC-EV were characterized through electron microscopy, nanoparticle tracking analysis, and immunoblotting[7]. The HBMSC-EV were visualized with electron microscopy (FEI Morgagni 268) after fixation and contrasted in 4% uranyl acetate. The size, number, and distribution of the HBMSC-EV were quantified with the Nanosight NS500 (Malvern Instruments). 10 µg of HBMSC-EV protein was loaded in a Bis-Tris gel and run using MOPS-SDS running buffer. The following markers were evaluated through immunoblotting: CD81 (Cell Signaling #52892S, 1:1,000), CD9 (Cell Signaling #13403S, 1:1,000), Alix (Cell Signaling #92880S, 1:1,000), GAPDH (Cell Signaling #97166S, 1:1,000), heat shock protein 70 (HSP70) (Cell Signaling #4872T, 1:1,000), and albumin (Cell Signaling #4929S, 1:1,000)[11].
Animals
Female and male FVB/NCrl mice (8-10 weeks old, Charles River Stock No. 207) were acclimatized and housed at the Coro Building Barrier facility. Experiments were carried out in accordance with the approved protocol via the Institutional Animal Care and Use Committee (Protocol 1844667/CMTT# 5017‐22).
Echocardiogram
Echocardiogram (Vevo 2100, FUJIFILM VisualSonic Inc.) was performed preoperatively, and postoperatively on postoperative days 3, 7, 14, 21, and 28. The mice were anesthetized with 2% isoflurane and monitored to ensure normothermia and heart rate between 400-600 beats per minute. Left heart systolic function was evaluated by obtaining two-dimensional parasternal long-axis views, and proximal, mid-papillary and distal short-axis views. Through Simpson’s method on Vevo Lab 5.6.0, left ventricular ejection fraction (LVEF) and fractional shortening (FS) were quantified.
Surgical procedure: left anterior descending (LAD) coronary artery ligation
Anesthesia was induced with 3% isoflurane and ketamine (100 mg/kg). Buprenorphine SR (1 mg/kg) was administered in the dorsal fat pad. The mice were intubated and ventilated (MiniVent Type 845, Harvard Apparatus) and maintained at 2% isoflurane. A left thoracotomy in the 3rd interspace was made to expose the heart. The LAD artery was ligated with an 8-0 nylon suture 2-3 mm below the left atrial appendage with resulting blanching and dyskinesia[12].
Immediately after ligation, the mice received one of the following injections [Figure 1]:
Figure 1. Wild-type mice underwent left anterior descending coronary artery (LAD) ligation to induce myocardial infarction, and then were split into a tail vein or intramyocardial injection group to receive either human bone mesenchymal stem cell-derived extracellular vesicles (HBMSC-EV) or a negative saline control. The mice were then allowed to recover postoperatively, and underwent postoperative echocardiogram to evaluate cardiac function. The mice were euthanized on postoperative day 28 for organ harvest. This image was created using BioRender.com and used with permission.
1. IM injection control (IM-C) (n = 8): 10 µL PBS with 1% DMSO.
2. IM injection HBMSC-EV (IM-EV) (n = 9): 2 × 109 HBMSC-EV particles in 10 µL PBS with 1% DMSO.
3. Tail vein injection control (IV-C) (n = 8): 10 µL PBS with 1% DMSO + 190 µL PBS.
4. Tail vein injection HBMSC-EV (IV-EV) (n = 8): 2 × 109 HBMSC-EV particles in 10 µL PBS with 1% DMSO + 190 µL PBS.
The dosage of 2 × 109 HBMSC-EV particles was chosen based on previous biodistribution studies that visualized positive organ uptake at this dosage 2 h after injection[7]. The intramyocardial injection was done with a Neuros Syringe (Hamilton, 1183U32) in two locations (5 µL per location) - immediately below the stitch in the ischemic myocardium and 1-2 mm lower in the anterior ischemic myocardium after clear myocardial blanching was visualized. The tail vein injection was done with a 0.5 mL insulin syringe. The thoracotomy was closed with absorbable sutures and the pneumothorax was evacuated. The mice were awakened from anesthesia, extubated, and allowed to recover in a warm cage.
On postoperative day 28, the mice were euthanized and the heart, lungs, liver, spleen, and kidneys were harvested.
Histology
The hearts and livers were embedded in Tissue-Tek OCT (Sakura Finetek) and were stored at -80 °C. Sectioning was done in 5 µm thickness. At the mid-papillary level, the heart was evaluated for infarct size and anterior border zone interstitial fibrosis with Masson-Trichrome staining. Quantification was done through ImageJ.
Given the previous positive hepatic uptake of HBMSC-EV after IV delivery, the liver tissues of the IV-C and IV-EV groups were further evaluated. To look for changes in liver inflammation, fibrosis and proliferation, hematoxylin and eosin, Masson-Trichrome and immunofluorescence staining of proliferating cell nuclear antigen (PCNA) (Cell Signaling #2586, 1:400) were performed, respectively. The hematoxylin and eosin images were examined for leukocyte infiltration. Fibrosis was quantified using ImageJ. Positive PCNA staining was determined via manual counting.
Immunoblotting
In a Bis-Tris gel, 10 μg of protein from liver tissue lysate was loaded and run in MOPS-SDS running buffer. The following markers were evaluated through immunoblotting: tumor necrosis factor alpha (TNF-α) (Cell Signaling #11948, 1:1,000), transforming growth factor beta (TGFβ) (Cell Signaling #3711, 1:1,000), SMAD 2/3 (Cell Signaling #8685, 1:1,000), mammalian target of rapamycin (mTOR) (Cell Signaling #2983, 1:1,000), B-cell lymphoma 2 (Bcl-2) (Cell Signaling #3498, 1:1,000), superoxide dismutase 1 (SOD1) (Cell Signaling #37385, 1:1,000), superoxide dismutase 2 (SOD2) (Cell Signaling #13141, 1:1,000), phospho-endothelial nitric oxide synthase (p-eNOS, Ser1177) (Cell Signaling #9570, 1:1,000), endothelial nitric oxide synthase (eNOS) (Cell Signaling #32027, 1:1,000), and beta-actin (Cell Signaling #58169, 1:1,000). The following secondary antibodies were used: anti-mouse IgG, HRP-linked antibody (Cell Signaling #7076, 1:5,000), and anti-rabbit IgG, HRP-linked antibody (Cell Signaling #7074, 1:5,000). The band intensities were normalized by beta-actin and quantified on ImageJ.
Statistical analysis
Continuous data were obtained from echocardiogram, histological, and immunoblotting results. Data analysis was performed on GraphPad Prism 9.0.2. The Shapiro-Wilk test was done to check for normality. For pairwise comparisons, either the unpaired t-test (parametric data) or the Mann-Whitney test (non-parametric data) was used. For comparisons of more than two groups, the one-way ANOVA (parametric) or Kruskal-Wallis (non-parametric) tests were used, followed by the post-hoc Tukey (parametric) or Dunn (non-parametric) tests.
RESULTS
No long-term improvements in cardiac recovery were found after HBMSC-EV injection, when delivered either IM or IV
Preoperatively, all mice had normal cardiac function on echocardiogram. Overall, there were no significant changes in either LVEF or FS by postoperative day 28 [Figure 2]. The FS of IM-EV was significantly greater than that of IV-EV on postoperative 7 (P = 0.0038), but these differences disappeared with time.
Figure 2. There were no significant long-term benefits in left ventricular systolic function. (A) There were no differences in left ventricular ejection fraction (LVEF) or fractional shortening (FS) on postoperative day 3. (B) There were no differences in LVEF on postoperative day 7, but the FS of IM-EV was significantly greater than that of IV-EV (P = 0.0038). (C) On postoperative day 28, there were no differences in either LVEF or FS; IM-C, IM control injection group (n = 8); IM-EV, IM extracellular vesicles injection group
Quantification of myocardial infarct after Masson-Trichrome staining showed that although there was a trend in differences in infarct size among the four groups, infarct size differences did not reach significance by IM or IV administration of HBMSC-EV (P = 0.05620) [Figure 3A]. There were also no significant changes in border zone interstitial fibrosis (P = 0.6333) [Figure 3B].
Figure 3. There were no significant changes in myocardial infarct size or border zone interstitial fibrosis after either IM or IV human bone mesenchymal stem cell-derived extracellular vesicle (HBMSC-EV) injection. (A) The infarct percentages of all four groups were not found to be significantly different (P = 0.05620). Representative images of the infarct size in each of the four groups are shown. Scale bar = 1 mm. (B) Anterior border zone interstitial fibrosis was not found to be significantly different (P = 0.6333).
Intravenously delivered HBMSC-EV do not affect hepatic inflammation, fibrosis or proliferation in the absence of liver disease
Hepatic uptake of IV HBMSC-EV after murine MI was demonstrated in previous studies, but this current study did not demonstrate any long-term effects of the HBMSC-EV in the absence of liver injury
Figure 4. Intravenous (IV) injection of human bone mesenchymal stem cell-derived extracellular vesicles (HBMSC-EV) did not alter hepatic inflammatory status, fibrosis, or proliferation. (A) Hematoxylin and eosin staining, Masson-Trichrome staining, and proliferating cell nuclear antigen (PCNA) immunofluorescence did not demonstrate differences in leukocyte infiltration, fibrosis, and cellular proliferation, respectively. Scale bar = 100 µm. (B) Quantification of % fibrosis on Masson-Trichrome staining was not significantly different (P = 0.8167) between the IV control (IV-C) and IV HBMSC-EV (IV-EV) injection groups. Statistical analysis was performed using the Shapiro-Wilk and unpaired t-test. (C) Immunoblotting showed no differences in major regulators of inflammatory and fibrosis pathways, such as tumor necrosis factor-alpha (TNF-α), transforming growth factor-β (TGF-β), and SMAD 2/3. Cell proliferation regulator mammalian target of rapamycin (mTOR) expression was increased in the IV-EV group, but this finding is difficult to interpret given the complexity of mTOR regulation and the negative PCNA findings. Oxidative protein expression changes are difficult to interpret as well, given the lack of hepatic oxidative injury in this model.
Concurrent immunoblotting of major signaling proteins of inflammatory and fibrosis and proliferative pathways supported the histological results [Figure 4C]. Expression of tumor necrosis factor-alpha (TNF-α), a major regulator of the inflammatory process, and some of the key fibrotic pathway markers transforming growth factor-β (TGF-β) and SMAD 2/3 was not changed after IV HBMSC-EV (P = 0.3944, P = 0.1961, respectively). The mammalian target of rapamycin (mTOR) is involved with cellular growth and had increased expression (P = 0.0066) after HBMSC IV injection, but given the complexity of its regulation, this finding is difficult to interpret. B-cell lymphoma 2 (Bcl-2), an apoptosis regulator, was not significantly different (P = 0.8055). Proteins involved in oxidative stress were evaluated as well, and although expression of superoxide dismutase 1 (SOD1) and total endothelial nitric oxide synthase (eNOS) were altered after HBMSC-EV injection (P < 0.0001, P = 0.0334, respectively), these findings are difficult to interpret since there was no hepatic oxidative injury in this model.
DISCUSSION
The findings of this study clearly show that a single dose of either IM or IV HBMSC-EV injection does not improve cardiac outcomes significantly after acute MI. It is intriguing that although previous studies have shown cardiac benefits with IM injection (2, 4, 5, 13), the current study did not. There may be several reasons for our findings in the present study. Acute MI represents a severe form of cardiac injury where our single dose injection of HBMSC-EV may not be sufficient to achieve a meaningful effect. Our dose was
Secondly, how should studies showing improvement in cardiac function after systemic administration of EVs be interpreted when no significant cardiac uptake of EVs has ever been shown? One study systemically injected a porcine model of acute MI (via left circumflex artery ligation) with a 1,000 µg of mesenchymal stem cell-derived EV protein twice daily for 7 days following MI, and they achieved a clear reduction of infarct size (30%-40%) on cardiac MRI[3]. Cardiac MRI is a highly accurate modality and thus the improvements in cardiac function can be believed. However, one significant caveat to this study is the massive dose of the EVs used, which may not be practical given the vast quantity of cells, labor, supplies, and equipment needed to produce EVs - this would make the clinical use of EVs highly expensive and time-consuming for any benefit to be achieved. It is definitely possible that some EVs are taken up in the heart, as the current EV biodistribution detection methods such as fluorescent molecular tomography are not very sensitive, in that smaller IM EV doses are not detected[7]. Thus, if EVs are to be successfully transitioned to clinical use, more refined administration techniques will be needed or the cardiac-homing properties of EVs will need to be amplified.
In conclusion, a single-dose administration of neither IM nor IV injection of 2 × 109 HBMSC-EV particles in a murine myocardial infarction model resulted in significantly improved cardiac function. This necessitates studies aimed at improving cardiac delivery by developing bioengineered EVs with cardiac or organ-specific delivery properties. The main limitation of this study is the lack of optimization of HBMSC-EV dose testing to see if increased doses or multiple doses could result in a post-MI cardiac benefit. However, the current study suggests that in order to treat cardiovascular disease efficiently in clinical settings - future studies are required to optimize EV dosage and administration routes, and/or bioengineer EVs to render cardiac-homing properties.
DECLARATIONS
Authors’ contributions
Conceptualization, methodology: Xu CM, Teixeira RB, Abid MR
Formal analysis: Xu CM, Faherty P, Abid MR
Funding acquisition: Sellke FW, Abid MR
Investigation: Xu CM, Broadwin M, Faherty P, Sabra M
Project administration: Xu CM, Abid MR
Supervision: Abid MR
Validation, writing - original draft preparation: Xu CM
Visualization: Xu CM, Faherty P
Writing - review & editing: Xu CM, Broadwin M, Faherty P, Teixeira RB, Sabra M, Sellke FW, Abid MR
Availability of data and materials
Upon request from the first author, Xu CM.
Financial support and sponsorship
Funding for this research was provided by the National Heart, Lung, and Blood Institute (NHLBI) 1R01HL133624 (Abid MR), 2R56HL133624 (Abid MR), and by the Rhode Island Foundation Grant 1472420231352 (Abid MR); R01HL46716 and R01HL128831-01A1 (Sellke FW). Xu CM was supported by T32 GM065085 (Albina J). Broadwin M was supported by T32 HL160517 (Sellke FW).
Conflicts of interest
All authors declared that there are no conflicts of interest.
Ethical approval and consent to participate
Not applicable.
Consent for publication
Not applicable.
Copyright
© The Author(s) 2023.
REFERENCES
1. Xuan L, Fu D, Zhen D, et al. Extracellular vesicles derived from human bone marrow mesenchymal stem cells protect rats against acute myocardial infarction-induced heart failure. Cell Tissue Res 2022;389:23-40.
2. Potz BA, Scrimgeour LA, Pavlov VI, Sodha NR, Abid MR, Sellke FW. Extracellular vesicle injection improves myocardial function and increases angiogenesis in a swine model of chronic ischemia. J Am Heart Assoc 2018;7:e008344.
3. Charles CJ, Li RR, Yeung T, et al. Systemic mesenchymal stem cell-derived exosomes reduce myocardial infarct size: characterization with MRI in a porcine model. Front Cardiovasc Med 2020;7:601990.
4. Gallet R, Dawkins J, Valle J, et al. Exosomes secreted by cardiosphere-derived cells reduce scarring, attenuate adverse remodelling, and improve function in acute and chronic porcine myocardial infarction. Eur Heart J 2017;38:201-11.
5. Scrimgeour LA, Potz BA, Aboul Gheit A, et al. Extracellular vesicles promote arteriogenesis in chronically ischemic myocardium in the setting of metabolic syndrome. J Am Heart Assoc 2019;8:e012617.
6. Scrimgeour LA, Potz BA, Aboul Gheit A, et al. Intravenous injection of extracellular vesicles to treat chronic myocardial ischemia. PLoS One 2020;15:e0238879.
7. Xu CM, Sabe SA, Brinck-Teixeira R, Sabra M, Sellke FW, Abid MR. Visualization of cardiac uptake of bone marrow mesenchymal stem cell-derived extracellular vesicles after intramyocardial or intravenous injection in murine myocardial infarction. Physiol Rep 2023;11:e15568.
8. Kang M, Jordan V, Blenkiron C, Chamley LW. Biodistribution of extracellular vesicles following administration into animals: a systematic review. J Extracell Vesicles 2021;10:e12085.
9. Wang C, Zhou H, Wu R, et al. Mesenchymal stem cell-derived exosomes and non-coding RNAs: regulatory and therapeutic role in liver diseases. Biomed Pharmacother 2023;157:114040.
10. Xu CM, Karbasiafshar C, Brinck Teixeira R, et al. Proteomic assessment of hypoxia-pre-conditioned human bone marrow mesenchymal stem cell-derived extracellular vesicles demonstrates promise in the treatment of cardiovascular disease. Int J Mol Sci 2023;24:1674.
11. Théry C, Witwer KW, Aikawa E, et al. Minimal information for studies of extracellular vesicles 2018 (MISEV2018): a position statement of the international society for extracellular vesicles and update of the MISEV2014 guidelines. J Extracell Vesicles 2018;7:1535750.
12. Reichert K, Colantuono B, McCormack I, Rodrigues F, Pavlov V, Abid MR. Murine left anterior descending (LAD) coronary artery ligation: an improved and simplified model for myocardial infarction. J Vis Exp 2017;122:55353.
13. Li H, Ding J, Liu W, et al. Plasma exosomes from patients with acute myocardial infarction alleviate myocardial injury by inhibiting ferroptosis through miR-26b-5p/SLC7A11 axis. Life Sci 2023;322:121649.
Cite This Article
Export citation file: BibTeX | RIS
OAE Style
Xu CM, Broadwin M, Faherty P, Teixeira RB, Sabra M, Sellke FW, Abid MR. Lack of cardiac benefit after intramyocardial or intravenous injection of mesenchymal stem cell-derived extracellular vesicles supports the need for optimized cardiac delivery. Vessel Plus 2023;7:33. http://dx.doi.org/10.20517/2574-1209.2023.98
AMA Style
Xu CM, Broadwin M, Faherty P, Teixeira RB, Sabra M, Sellke FW, Abid MR. Lack of cardiac benefit after intramyocardial or intravenous injection of mesenchymal stem cell-derived extracellular vesicles supports the need for optimized cardiac delivery. Vessel Plus. 2023; 7: 33. http://dx.doi.org/10.20517/2574-1209.2023.98
Chicago/Turabian Style
Xu, Cynthia M., Mark Broadwin, Patrick Faherty, Rayane Brinck Teixeira, Mohamed Sabra, Frank W. Sellke, M. Ruhul Abid. 2023. "Lack of cardiac benefit after intramyocardial or intravenous injection of mesenchymal stem cell-derived extracellular vesicles supports the need for optimized cardiac delivery" Vessel Plus. 7: 33. http://dx.doi.org/10.20517/2574-1209.2023.98
ACS Style
Xu, CM.; Broadwin M.; Faherty P.; Teixeira RB.; Sabra M.; Sellke FW.; Abid MR. Lack of cardiac benefit after intramyocardial or intravenous injection of mesenchymal stem cell-derived extracellular vesicles supports the need for optimized cardiac delivery. Vessel Plus. 2023, 7, 33. http://dx.doi.org/10.20517/2574-1209.2023.98
About This Article
Special Issue
Copyright
Data & Comments
Data
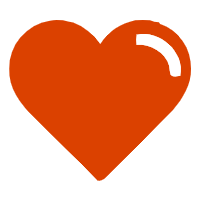

Comments
Comments must be written in English. Spam, offensive content, impersonation, and private information will not be permitted. If any comment is reported and identified as inappropriate content by OAE staff, the comment will be removed without notice. If you have any queries or need any help, please contact us at support@oaepublish.com.