Strategies for prevention of spinal cord ischemia in the management of thoracic and thoracoabdominal aneurysms
Abstract
Spinal cord ischemia (SCI) is undoubtedly the most devastating adverse event that occurs after either a thoracic aortic aneurysm (TAA) or thoracoabdominal aortic aneurysm (TAAA) repair. While open surgery techniques and minimally invasive endovascular options are now available for treating complex anatomy aortic aneurysms, spinal cord ischemia still occurs to a greater extent than desirable. Multiple risk factors have been associated with this adverse event, such as advanced age, perioperative hypotension, extent of the repair, and ligation of multiple intercostal and lumbar arteries during the surgical repair. The present literature review aims to analyze the contributing risk factors for SCI in the context of aortic surgery, explore the most relevant strategies for preventing postoperative SCI, and discuss the current management strategy when this complication occurs.
Keywords
INTRODUCTION
The incidence of thoracic aortic aneurysms is estimated at 6 cases per 100,000 per year[1], 40% of them involving the descending thoracic aorta (TAA) and 10% the thoracoabdominal aorta (TAAA)[2].
INCIDENCE OF SCI AFTER TAA AND TAAA REPAIR
The open surgery techniques have substantially evolved since the successful repairs of TAA and TAAA, as reported by DeBakey, CooleyField[7,8], and EtheredgeField[9]. However, the risk of spinal cord ischemia remains significant [Table 1].
Incidence of SCI reported in the current literature, considering different extensions of aortic repair performed either open or endovascularly
Author | Type of procedure | Number of patients included in the study | Reported incidence of SCI | |
Open surgery | Nishi et al.[10] | Open total arch replacement | 61 | 6.6% |
Chiesa et al.[11] | Open repair of descending thoracic aneurysms | 194 | 4.6% | |
Coselli et al.[12] | TAAA open repair | 3,309 | 4%-13.9% | |
Endovascular procedures | Liang et al.[13] | Endovascular arch repair | 40 | 2.5% |
Matsumura et al.[14] | Thoracic endovascular aneurysm repair (TEVAR) | 160 | 5.6% | |
Motta et al.[15] | Fenestrated/branched repair (F/BEVAR) of complex anatomy aortic aneurysms | 150 | 2.6% | |
Aucoin et al.[16] | Fenestrated/branched repair (F/BEVAR) of complex anatomy aortic aneurysms | 1,681 | 7.1% |
Initially indicated only for patients with numerous comorbidities who were not suitable candidates for open procedures, endovascular repair has become the approach of choice in the treatment of complex anatomy aneurysms at centers of excellence[17], and is currently recommended by the Clinical Practice Guidelines published by the European Society for Vascular Surgery in 2019[18]. Prior published studies indicate that the risk of SCI associated with aortic surgery ranges from 3%-14% [Table 1].
SPINAL CORD BLOOD SUPPLY REVIEW
The spinal cord arterial supply is composed of a complex network connecting multiple vessels responsible for its blood supply, such as the vertebral arteries (VA), the intercostal and lumbar arteries, and the hypogastric arteries. Biglioli et al., in an experimental study with cadavers, described the collateral pathways that contribute to spinal cord perfusion[19]. Three arteries run longitudinally along the length of the spinal cord, including one anterior spinal artery (ASA) and two posterior spinal arteries (PSA). The anterior spinal artery originates by the level of the foramen magnum and is responsible for the irrigation of the two anterior thirds of the spinal cord. The two posterior spinal arteries (PSA) originate either from the vertebral arteries or posterior inferior cerebellar arteries[20]. The spinal arteries are primarily fed by the subclavian and vertebral arteries in the cervical segment of the spine. Segmental arteries in the thoracic and lumbar regions provide additional blood supply to the spinal arteries[21], and the Adamkiewicz artery, which is the largest anterior segmental vessel, branching off the left side of the distal thoracic or proximal abdominal aorta between T8 and L2 in 75% of people. The primary blood flow supply for the distal spinal cord and cauda equina comes from the hypogastric arteries and their branches[22]. The pial network, which covers the entirety of the spinal cord, allows communication between the anterior and posterior spinal arteries[23].
More recently, Griepp et al. introduced the collateral network concept, which encompasses the existence of an extensive network of arteries that supply blood to the cord and paraspinal muscles, presenting the unique capability of adapting if faced with interruption[24]. It has been demonstrated that after interruption of segmental arteries[24], an early vasodilation of the ASA is observed, followed by a definitive increment in the size and density of small arterioles associated with modifications in the direction of the blood flow. This later enables blood flow in a cranial or caudal direction from the hypogastric or subclavian arteries as needed. Finally, the concept of vascular territories organizes the spinal cord perfusion in four arterial supply territories: cervical arteries (fed mainly by the subclavian and vertebral arteries), intercostal arteries, lumbar arteries, and hypogastric arteries[25]. These territories can communicate through the aforementioned massive collateral network in the occasion of segmental artery occlusion.
PATHOPHYSIOLOGY & CLINICAL/SURGICAL RISK FACTORS FOR SCI IN THE CONTEXT OF AORTIC SURGERY
In the past, interruption of the artery of Adamkiewicz was considered the primary cause of SCI after aortic surgery. This theory has become less popular with the emergence of the collateral network concept. Other causes implicated in the multifactorial genesis of SCI have been extensively analyzed in literature [Table 2]. Regardless of the type of aortic repair performed, the collateral network initiates the compensatory mechanisms after the interruption of the feeding arteries to the spinal cord occurs, so the collateral supply can adapt to the loss of feeding segmental vessels. When this mechanism is insufficient, SCI can occur.
Main risk factors that contribute to the pathophysiology of SCI in the context of thoracic and thoracoabdominal aneurysms surgery
Demographics: age above 70 years, renal function impairment, hypertension, chronic obstructive pulmonary disease, and diabetes mellitus[26,27] |
Aortic cross-clamping and unclamping: produces hypertension proximal to the clamp, increased central venous pressure, and distal hypotension, which combined, produce spinal cord ischemia[28,29]. Prolonged cross-clamping[30] increases the risk of SCI even more |
Other surgical risk factors: Extent II TAAA[27], emergent presentation with a ruptured aneurysm, presence of dissection[30], prior or concomitant AAA surgery, the extension of the aortic repair, excessive intraoperative blood loss, long duration of the procedure, and coverage of the subclavian and hypogastric arteries, especially if more than two vascular independent territories will be compromised[31] |
Extensive aortic coverage in endovascular procedures: Produces obliteration of multiple segmental arteries[31], which is specifically concerning during endovascular repair because intercostals cannot be surgically reimplanted[32,33] |
Perioperative hypotension[34-36]: with mean arterial pressure < 70 mmHg |
Presence of lower density mural thrombus/plaque in the descending thoracic aorta: risk factor for microembolism[37] |
Atheroemboli[38] |
occlusion of spinal cord feeding vessels with air[39] |
CLINICAL MANIFESTATIONS OF SCI AFTER TAA AND TAAA REPAIR
As for the clinical presentation, SCI symptoms can range from mild muscle weakness to paraplegia[40]. The symptoms may develop immediately as observed by Spanos et al. or in a delayed fashion as reported by Alizadegan et al. and components of the autonomic nervous system may become involved[6,41,42].
PREOPERATIVE PLANNING
Subclavian artery patency relevance
As previously mentioned, the subclavian arteries significantly contribute to spinal cord perfusion. In most cases, the vertebral arteries branch off the posterosuperior aspect of the first segment of the subclavian arteries, bilaterally[43], and therefore the subclavian artery patency is a crucial piece of information during the planning of the surgical treatment of TAA and TAAA. Moreover, in aortic aneurysms affecting zones 2 and 3[44] of the aortic arch[45], the repair would compromise the origin of the left subclavian artery (LSA). The current medical literature is inconclusive concerning LSA revascularization prior to a TEVAR.
Conversely, other studies report that LSA coverage is well tolerated during TEVAR procedures, even without revascularization. In a retrospective multicenter study including 1,189 patients submitted to TEVAR for the treatment of TAA, Maldonado et al. concluded that LSA revascularization was not protective against SCI, and reported an increased risk of cerebrovascular accidents in female patients submitted to LSA revascularization[50]. Riesenman et al., in a retrospective analysis including 112 TEVAR patients, did not report SCI events among the 24 cases with endograft implanted in zone 2, even though none of them underwent LSA revascularization[51]. Coverage of the LSA is contraindicated without prior revascularization in situations such as the presence of a dominant left vertebral artery[51]. It is also critical to evaluate the contribution from the circle of Willis’ anatomy during the surgical planning of procedures that involve the subclavian arteries. Table 3 summarizes the SVS recommendations for LSA revascularization in the context of TEVAR with LSA coverage.
SVS Practice Guidelines (2010)[52]: recommendations of LSA revascularization for TEVAR patients with LSA coverage (GRADE 1, level C)
Left vertebral dominance[51] (absent, atretic, or occluded right vertebral artery, absence of communication between the left VA and the circle of Willis with termination of the left VA artery into the posterior inferior cerebellar artery) |
Compromised collateral spinal cord perfusion |
Prior ligation of lumbar and middle sacral arteries during infrarenal aortic surgery |
Planned coverage of the descending thoracic aorta longer than 200 mm |
Hypogastric artery occlusion |
Prior left internal mammary-to-coronary artery bypass |
Hypogastric artery patency relevance
The patency of the hypogastric arteries plays a vital role in the context of SCI risk factors, especially in patients submitted to extensive aortic repair, as these arteries significantly contribute to the perfusion of the distal spinal cord[53]. Picone et al., described seven patients who developed spinal cord ischemia after abdominal aortic surgeries, five of whom had unilateral or bilateral hypogastric occlusion during the procedures[54]. Eagleton et al., in a study including TEVAR (n = 201), FEVAR (n = 227), BEVAR (n = 472), and EVAR (351), reported an incidence of SCI of 2.8% (n = 36)[55]. Of these affected patients, fourteen had preoperative unilateral or bilateral hypogastric occlusion, and seven had coverage of at least one hypogastric artery during the endovascular procedure, highlighting the relevance of the internal iliac arteries patency in the overall risk of SCI.
The importance of preserving hypogastric perfusion cannot be stressed enough. For over a decade now, iliac branched grafts have been used as a safe and successful strategy of antegrade blood flow preservation to the hypogastric arteries during aortic surgery in those cases with aneurysmal degeneration affecting the iliac bifurcation[56]. Simonte et al. reported no occurrence of spinal cord ischemia in a study including 157 consecutive cases in which iliac branch devices were utilized[57]. Schneider et al., in a study on long-term outcomes of iliac branch endografts, reported primary patency of 95.1% of the internal iliac artery limb and freedom of secondary intervention of 90.5% at the 5-year landmark, demonstrating that the iliac branch grafts are a valuable tool in the preservation of the hypogastric artery flow[58].
The importance of the repair extension and intercostal/lumbar arteries coverage
Covering an extensive segment of the thoracoabdominal aorta could compromise intercostal and lumbar contribution to the spinal cord, and increase the risk of SCI. Feezor et al., in a study including 326 TEVAR patients, observed an incidence of 10% of SCI and concluded that patients who developed permanent deficits had a higher length of aortic coverage[59]. Flores et al., in a study including 25 patients undergoing TAA repair with the stented elephant trunk technique, reported that the combination of a distal landing zone below T7 and a history of prior AAA repair was the strongest predictor for the occurrence of SCI, with an odds ratio of 5.46 (P = 0.0047)[60]. Czerny et al. observed that simultaneous obliteration of at least two independent vascular territories associated with intraoperative hypotension is a significant risk factor for SCI[61]. In a study including 1096 TAA and TAAA cases submitted to open repair, Afifi et al. reported that ligation of intercostal arteries between the T8 and T12 significantly increased the risk of paraplegia[62]. The authors report that reimplantation of intercostal arteries only minimally increases the procedure duration. Therefore, this would potentially reduce the risk of SCI without significantly impacting the overall procedure risk. Lastly, it has been reported that total aortic coverage longer than 205 mm is associated with the development of SCI[63,64].
Staged strategy
Especially for complex TAA and TAAA that would require an extended coverage of the thoracic and abdominal aorta, the staged strategy is an essential tool in preventing SCI, either for open or endovascular repairs. The rationale behind this approach consists of dividing the repair into two shorter and less morbid interventions, so the collateral network has enough time to compensate for the reduction of blood flow, potentially diminishing the risk of postoperative SCI. Etz et al., in a study including 90 TAAA patients submitted to open procedures, observed a significantly lower rate of paraparesis and paraplegia after staged TAAA repair compared to non-staged patients[65]. Interestingly, this result was observed despite a significantly higher number of intercostal and lumbar arteries being sacrificed in the staged group. In a study including 87 TAAA patients submitted to endovascular repair, O’Callaghan et al. observed a significantly lower incidence of SCI in patients undergoing staged repair compared to patients who underwent non-staged repair, even though the length of aortic coverage was significantly greater in the staged group[66]. Moreover, all the SCI symptoms in the staged group were temporary. A recent publication by Dias-Neto et al., including 1,947 patients from the International Aortic Research Consortium, demonstrated that the staged strategy significantly reduced the risk of permanent paraplegia after fenestrated/branched repair of extension I-III TAAA[67]. This body of evidence highlights the potential for SCI risk reduction of the staged strategy, either for open or endovascular repair of TAAA.
Minimally invasive segmental artery coil embolization
Minimally invasive segmental artery coil embolization (MISACE) is a technique aimed to provide a preconditioning to ischemia prior to the TAA or TAAA repair. The rationale behind this strategy is to improve spinal cord vascularization through neo-angiogenesis before the index procedure, either open or endovascular. Geisbush et al. demonstrated in a swine model that the coiling of 2-4 segmental arteries significantly prevented paraplegia after extensive thoracoabdominal aneurysm repair[68]. Etz et al. described the first-in-man successful experience with this technique performed in two patients: one planned for an open repair of a type III TAAA, and the second planned for an endovascular repair of a type II TAAA[69]. Both patients developed no neurologic injuries either after MISACE or the TAAA repair. Branzan et al., in a study including 57 TAAA cases reported the embolization of 77.7% of the segmental arteries and no occurrence of SCI using the MICACE technique[70]. According to a study published by Addas et al., the imaging planning prior to the MISACE technique consists of observing anatomical landmarks close to the site of segmental arteries’ origin to assist in selecting target arteries during the procedure[71]. Preoperative images also guided coil sizing, fluoroscopy positioning and angulation. The authors utilized the transfemoral approach in all the 17 patients included in their study. Once the target vessel was selected, the coils were deployed in the proximal section of the chosen segmental artery, proximal to its branching point. The mean time window between the MISACE and the TAAA repair was 51.2 days (5-110 days).
Although the studies mentioned above provide encouraging results, numerous complications can occur in the context of the MISACE technique, such as the incomplete occlusion of the targeted segmental arteries usually associated with the use of antiplatelet therapy or anticoagulants, loss of coils in the aorta, and the most severe of all, SCI. In addition, anatomical challenges related to the segmental arteries, such as large vessel diameter and tortuosity, and the long duration of these procedures associated with high contrast volume administration should be considered before the application of the MISACE technique[71]. A clinical trial that started in Europe in November 2018, with a completion date estimated for March 2023, will assess the clinical safety and efficacy of the MISACE procedure[72].
PERIOPERATIVE STRATEGIES FOR SCI PREVENTION: LUMBAR DRAIN UTILIZATION, THE ROLE OF NEUROMONITORING AND INTRAOPERATIVE HEMODYNAMICS MANAGEMENT
Spinal drain utilization as a strategy for SCI prevention
The perfusion of the spinal cord relies on numerous factors including mean arterial pressure (MAP), cerebrospinal fluid pressure (CSFP), and central venous pressure (CVP. Spinal cord perfusion pressure (SCPP) is directly proportional to the MAP and inversely proportional to the CSFP and CVP, corresponding to the neuraxial outflow pressure[31]. Coselli et al., in a randomized controlled trial including 145 TAAA patients submitted to open repair, demonstrated an 80% relative risk reduction of postoperative deficits in the group with CSF drain placed preoperatively[73]. Suarez-Pierre et al., in a retrospective analysis of 4,287 patients included in the TEVAR module of the Vascular Quality Initiative (VQI), concluded that preoperative spinal drainage placement significantly reduced the risk of SCI[74].
However, Aucoin et al., in a study including eight of the Principal Investigators of the US Aortic Research Consortium (US-ARC)[75,76], discuss that the indications of prophylactic CSF drain placement are evolving due to potentially severe complications related to drain placement. These authors recommend the placement of a preoperative drain in Type I-III TAAA cases, in patients with prior history of aortic infrarenal repair, cases with “shaggy aorta”, hypogastric artery abnormalities (unilateral occlusion or bilateral stenosis), or abnormalities in the vertebral arteries. Our current practice is changing to therapeutic drain only.
As for the complications related to spinal drain placement, Kärkkäinen et al., in a study including 187 patients submitted to 240 procedures (F/BEVAR or first-stage TEVAR) with the prophylactic placement of CSF drain, observed 6% of intracranial hypotension and 3% of spinal hematomas resulting in paraplegia or transient paraparesis in 2%[77]. A meta-analysis conducted by Rong et al. observed a polled event rate of 6.5% for overall CSF drainage-related events and 2.5% for CSF drainage-related severe complications such as intracranial hemorrhage, meningitis, epidural hematoma, and neurological deficits[78].
In light of the described complications, in some institutions, a therapeutic strategy is being considered the best option in F/BEVAR cases[75], using perioperative neuromonitoring to detect SCI before the onset of symptoms postoperatively. In some institutions, prophylactic spinal drains are placed only in high-risk situations, such as patients with preoperative bilateral hypogastric occlusion requiring extensive repair, or cases with prior neurological deficits. More recently, the US-ARC has advocated a therapeutic-drain-only approach to patients undergoing endovascular TAAA repair.
Hemodynamic management for spinal cord protection during TAA And TAAA repair
To promote appropriate perfusion and oxygen delivery to the spinal cord during TAA and TAAA repair, a cardiac index (CI) above 2.5 L/min/BSA is recommended[79]. Attention is needed to avoid attempts of CI increment based on excessive volume infusion or on the excessive use of vasoactive drugs. The former could provoke elevation of the central venous pressure, which is inversely proportionate to the spinal perfusion pressure. The latter could produce undesirable vasoconstriction in the spinal cord microcirculation[80].
As for blood pressure management, intraoperative hypotension is a well-known risk factor for SCI after TAA and TAAA repair[81]. Aucoin et al., in the aforementioned study on the SCI prevention practices adopted by the US-ARC, report that the authors recommend permissive hypertension starting before the index procedure[75]. It should be maintained in the first 2 to 4 postoperative weeks, which is especially relevant for patients that postoperatively presented reversible symptoms of SCI. To achieve this goal, angiotensin receptor blockers and angiotensin-converting enzyme inhibitors should be withheld preoperatively at least 48 h before surgery. As for the alfa- and beta-blockers, there was no consensus among the PIs about discontinuing or not these medications, and in most cases, the beta-blockers were continued throughout the perioperative period. The spinal cord perfusion pressure (SCPP) is directly proportionate to mean arterial pressure[82], hence the hazardous effect of hypotension over the SCPP. A goal of SCPP > 80 mmHg is recommended. To fulfill this goal, a MAP > 90 mmHg is desired, and in cases in which a lumbar drain has been placed preoperatively, the targeted CSF pressure should be kept at
Optimizing hemoglobin levels perioperatively also plays a significant role in the SCI strategy. Behzadi et al., in a study including 174 TAA and suprarenal AAA patients submitted to either open or endovascular repair, observed that preoperative hemoglobin < 9 mg/dL was a risk factor for the occurrence of SCI[83]. Perioperative hemoglobin ≥ 10 mg/dL has been reported in the literature as part of protocols for SCI prevention in TAAA patients submitted to F/BEVAR[84].
Another valuable tool for spinal cord protection during aortic surgery is epidural cooling for regional spinal cord hypothermia. Tabayashi et al., in a study including 37 patients submitted to open thoracic or thoracoabdominal aorta replacement for TAAA or aortic dissection, concluded that epidural cooling is a safe method of effectively reducing postoperative SCI[85]. The authors utilized a catheter placed into the epidural space to infuse precooled saline. The epidural cooling was initiated 30 min before aortic clamping, maintaining the temperature at 25 °C. Moreover, Bobadilla et al. suggest that mild intraoperative hypothermia, keeping body temperature between 32-35 °C, could improve spinal cord ischemic tolerance and aid in preventing SCI[86].
As for the pharmacological options that would potentially help, the use of mannitol (18% mannitol, 0.5 g/kg, infusion rate: 300 mL/h) can contribute to reducing the CSF pressures, as previously demonstrated in the literature[87,88]. Among the Principal Investigators of the Aortic Research Consortium, doses between 12.5 and 25 g of mannitol have been used intraoperatively during F/BEVAR procedures[75]. An experimental study also suggests that mannitol can function as a free radical scavenger; therefore, it would potentially contribute to reducing the risk of spinal cord ischemic injury in the context of TAA and TAAA repair. Naloxone is another drug that could be favorable in this context. Kunihara et al. observed that the intraoperative administration of this medication (1 microg/kg/h) has been shown to reduce the levels of excitatory neurotransmitters in the CSF, such as glutamate, which is significantly elevated in patients that presented SCI after TAA and TAAA repair, and can be considered an independent predictor of SCI in the context of aortic surgery[89].
Perioperative glucose monitoring has been shown to decrease the risk of neurological deficits related to spinal cord ischemia. Hiramoto et al., in a study involving individuals who underwent branched endovascular aneurysm repair (BEVAR), reported a reduced incidence of postoperative lower extremity weakness in patients who were prescribed a perioperative insulin infusion protocol. The authors suggest that tight glycemic control should be considered for patients who will be submitted to extensive aortic coverage and therefore are at higher risk of SCI[90].
Finally, the role of steroids deserves attention, as their actions on the recovery after SCI[91] have been extensively debated in the literature. Laschinger et al. observed encouraging results in an experimental model in which animals submitted to aortic cross-clamping were analyzed[92]. The ones that received methylprednisolone intraoperatively and a second dose postoperatively presented no clinical evidence of neurological deficit, contrasting with the 67% incidence of permanent paraplegia observed in a control group that underwent the same duration of cross-clamping but did not receive the drug. Prior publications on SCI prevention in the context of aortic surgery suggest the intraoperative administration of steroids as part of a protocol of SCI prophylaxis. Bobadilla et al., in a study including 94 patients submitted to TEVAR mainly for the treatment of aneurysms or dissections, reported an incidence of SCI as low as 1.1% and attributed these results to the implementation of a proactive spinal cord protective protocol, which included the administration of methylprednisolone 30 mg/kg[86]. Similarly, Acher et al. observed a very low incidence of SCI (0.65%) in 155 patients submitted to TEVAR, encouraging the simultaneous use of numerous spinal cord protective measurements, including the intraoperative administration of methylprednisolone
The role of neuromonitoring in the context Of TAA and TAAA repair
Intraoperative neuromonitoring (IONM) has become a valuable aid in the context of complex anatomy aortic aneurysm repair. As recent publications have demonstrated, it can support intraoperative decision-making, either during open or endovascular repair[36,95]. Motor-evoked potentials (MEP) monitor the integrity of the descending corticospinal pathway, whereas somatosensory-evoked potentials (SSEP) assess the functionality of the dorsal column somatosensory tract for proprioception and vibration[96].
Kolesár et al. experimentally demonstrated a region-specific sensitivity of the spinal cord to ischemia[97], reporting that motoneurons in the ventral horns are more sensitive to ischemia than neurons in other segments of the spinal cord, which can explain why MEP is more sensitive for SCI monitoring than SSEP. MEP are significantly reduced or not detectable within two minutes of acute ischemia[98], providing intraoperative real-time information to the surgeons and anesthesiologists. Bianchi et al., in a study including 100 patients submitted to TAAA repair, observed that the percentage of cases with postoperative SCI was significantly higher in those patients who presented irreversible MEP deterioration during the procedure compared to the ones with reversible deterioration[99]. The authors concluded that actions intended to reverse MEP deterioration seem to be worthwhile in the endeavor of reducing the SCI risk. It is essential to highlight that MEP and SSEP have some limitations, such as: (1) volatile anesthetics interfering with the amplitude of the SSEP; therefore, their use should be kept to a minimum so this IONM modality can be accurately used; (2) the limb ischemia produced by the introducers and sheaths used during the endovascular interventions can significantly interfere with the evoked potentials interpretation; and (3) MEP and SSEP are not able to differentiate between moderate and severe SCI[64,100]. The use of MEP or SSEP is recommended with a level B of evidence by a multi-society guideline on the management of patients with thoracic aortic disease[26].
Near-infrared spectroscopy (NIRS) is another IONM option in the context of aortic surgery. NIRS is a method for indirectly evaluating spinal cord oxygenation and perfusion, as it indicates the blood oxygen saturation in the tissue underneath the NIRS sensor[101]. Considering the collateral network concept, numerous connections exist between the intraspinal and paraspinal networks[102]. Therefore, multiple electrodes placed at the thoracic and lumbar levels on the surface of paraspinal muscles could provide an indirect assessment of the oxygenation and perfusion of the spinal cord[101]. It has the advantage of being a noninvasive modality that can be applied not only during the surgical procedure but also during the postoperative management in the intensive care unit. Despite the encouraging publications on the use of NIRS in the context of aortic surgery, Vanpeteghem et al. stated in a review study that there is no sufficient evidence available for a precise cutoff that should be considered for SCI[103]. No consensus has been established recommending the clinical applicability of NIRS in aortic surgery to date.
RESCUE STRATEGIES WHEN SCI IS DIAGNOSED IN THE PERIOPERATIVE PERIOD OF TAA AND TAAA REPAIR [Table 4]
SCI with intraoperative onset
During the TAA or TAAA repair, constant attention to the neuromonitoring data is crucial to prompt maneuvers intended to improve spinal cord perfusion. Decreased MEP or SSEP should immediately trigger actions to increase MAP > 90 mmHg, such as the careful infusion of volume and the use of vasopressors, as well as the increase in CSF drainage to keep CSFP < 10 mmHg in the high-risk patients who received a lumbar drain preoperatively. If persistent changes in IONM are observed, maneuvers such as reimplantation of intercostal arteries during open repair or temporary aneurysm sac perfusion (TASP) and modification of the sequence of implantation of components during endovascular approach could aid in increasing the blood flow to the spinal cord.
During open cases, the reimplantation of intercostal arteries has been demonstrated to be an effective tool in reducing the risk of SCI, without significantly increasing the duration of the procedure[62].
As for the rescue maneuvers during endovascular procedures, the rationale behind TASP is the prevention of the complete aneurysm sac thrombosis and maintenance of blood inflow to the spinal cord through intercostal and lumbar arteries achieved by intentionally leaving at least one target vessel non-scented. Kasprzak et al., in a study with 83 patients submitted to BEVAR for TAAA treatment[104], reported that the cases submitted to TASP had a significantly lower incidence of severe SCI or paraplegia compared to those that did not undergo this technique. These authors observed a median of 48 days (range 1-370 days) between the index procedure and completion of repair. Harrison et al. described an interval of 7-10 days between the TAAA repair and the complete exclusion of the aneurysm. TASP can also be obtained by leaving the contralateral limb of a bifurcated device incomplete[84,105].
Another intraoperative option to improve spinal cord perfusion during F/BEVAR for TAAA is the modification of the implantation sequence of the components during the repair. This maneuver aims to provide early pelvic and limb reperfusion by deploying the bifurcated component and iliac limbs and removing the large caliber sheaths from the iliac system before deploying the target vessel stents through an axillary access. Maurel et al., analyzed 204 TAAA patients submitted to endovascular repair and observed a significantly lower rate of SCI in patients who were submitted to early pelvic reperfusion using this technique[106].
Finally, in those cases presenting intraoperative SCI that did not have a spinal drain placed preoperatively, a therapeutic drain can also be indicated by the time the surgical intervention ends. According to the recommendations reported by Aucoin et al. on behalf of the US-ARC, keeping hemoglobin ≥ 10 mg/dL, MAP ≥ 90 mmHg, and obtaining an immediate image of the spine to rule out hematomas are also essential rescue maneuvers in this context[75].
The use of high-dose steroids has also been documented in this scenario in conjunction with the aforementioned techniques, but there is no consensus recommending this practice[107].
Rescue strategies
Intraoperative strategies | Keep MAP > 90 mmHg through careful infusion of volume and use of vasopressors |
Keep hemoglobin ≥ 10 mg/dL | |
If prophylactic spinal drain is in place, keep CSFP < 10 mmHg | |
Reimplantation of intercostal arteries (if open repair) open repair | |
TASP (in endovascular repair) | |
Modification of the sequence of implantation of components during endovascular repair | |
Postoperative strategies | Keep MAP > 90 mmHg through careful infusion of volume and use of vasopressors |
Keep hemoglobin ≥ 10 mg/dL | |
Therapeutic spinal drain (goal: CSFP < 10 mmHg) | |
Possible use of high-dose steroids |
SCI with postoperative onset
The multidisciplinary team must be attentive to the hemodynamic and neurologic status during the whole postoperative period of TAA and TAAA patients. A low threshold to trigger rescue strategies intended to prevent permanent neurologic deficits is recommended to reduce the recovery time from SCI in those presenting this adverse event. NIRS can be maintained in the intensive care unit for monitoring high-risk patients, although there is no consensus recommending its use and no sufficient evidence available for a precise cutoff that would be concerning for SCI.
The maneuvers described above should also be implemented for the cases with postoperative SCI. MAP above 90 mmHg and hemoglobin above 10 mg/dL are goals that should be pursued in these cases. If a prophylactic spinal drain was inserted, a therapeutic drain should be placed with the purpose of keeping CSFP below 10 mmHg. If the neurologic deficits do not improve as expected, lower CSFP values can be attempted as a heroic maneuver up to the discretion of the surgical team assisting the patient. However, in addition to the high risk of intracranial bleeding from aggressive CSF drainage, there is no consensus recommending this practice to date.
The use of therapeutic CSF drainage can also be indicated even in those cases presenting delayed-onset SCI symptoms, with satisfactory results reported in the literature[108]. Weissler et al. suggest that permissive hypertension (systolic blood pressure up to 150 mmHg) during the first month after TEVAR could also aid in the protection against SCI[109].
Finally, the use of high-dose steroids as part of a rescue protocol is described in the literature, even in cases with a delayed presentation of SCI[110], but there is no consensus recommending this practice.
CONCLUSION
Spinal cord ischemia prevention in the context of TAA or TAAA repair requires meticulous surgical planning including a thorough analysis of the contributions to spinal cord perfusion and repair extension, always evaluating the need for a staged approach. Careful perioperative strategies including hypotension avoidance and neuromonitoring, weighing the advantages and disadvantages of preoperative lumbar drain implantation are paramount in these cases. The strategies for preventing SCI discussed in this review can be applied in a combined fashion, depending on the patient’s anatomy and the planned repair. Vascular and cardiothoracic surgeons dedicated to the treatment of TAA and TAAA should be comfortable with all the SCI protective and rescue strategies in order to reduce the risk of this adverse event in this patient population.
DECLARATIONS
Authors’ contributions
Literature review; data interpretation; manuscript preparation; critical revision of the manuscript; approval of the manuscript: all authors.
Availability of data and materials
Not applicable.
Financial support and sponsorship
None.
Conflicts of interest
Vivian Carla Gomes: no disclosures; Federico Ezequiel Parodi: Stock options from Centerline Biomedical; Mark A Farber: WL Gore - Consulting, Clinical Trial Support; Getinge - Consulting; Cook - Research support, Clinical Trial support; ViTTA - Consulting, Clinical Trial support; Centerline Biomedical - Stock options, Clinical Trial support.
Ethical approval and consent to participate
Not applicable.
Consent for publication
Not applicable.
Copyright
© The Author(s) 2023.
REFERENCES
1. Ince H, Nienaber CA. Etiology, pathogenesis and management of thoracic aortic aneurysm. Nat Clin Pract Cardiovasc Med 2007;4:418-27.
3. Bickerstaff LK, Pairolero PC, Hollier LH, et al. Thoracic aortic aneurysms: a population-based study. Surgery 1982;92:1103-8.
4. Stoecker JB, Wang GJ. Epidemiology of thoracoabdominal aortic aneurysms. Semin Vasc Surg 2021;34:18-28.
5. Coselli JS, Green SY, Price MD, et al. Spinal cord deficit after 1114 extent II open thoracoabdominal aortic aneurysm repairs. J Thorac Cardiovasc Surg 2020;159:1-13.
6. Spanos K, Kölbel T, Kubitz JC, et al. Risk of spinal cord ischemia after fenestrated or branched endovascular repair of complex aortic aneurysms. J Vasc Surg 2019;69:357-66.
7. De Bakey ME, Cooley DA. Successful resection of aneurysm of thoracic aorta and replacement by graft. J Am Med Assoc 1953;152:673-6.
8. De Bakey ME, Cooley DA. Surgical treatment of aneurysm of abdominal aorta by resection and restoration of continuity with homograft. Surg Gynecol Obstet 1953;97:257-66.
9. Etheredge SN, Yee J, Smith JV, Schonberger S, Goldman MJ. Successful resection of a large aneurysm of the upper abdominal aorta and replacement with homograft. Surgery 1955;38:1071-81.
10. Nishi H, Mitsuno M, Tanaka H, Ryomoto M, Fukui S, Miyamoto Y. Spinal cord injury in patients undergoing total arch replacement: a cautionary note for use of the long elephant technique. J Thorac Cardiovasc Surg 2011;142:1084-9.
11. Chiesa R, Tshomba Y, Civilini E, et al. Open repair of descending thoracic aneurysms. HSR Proc Intensive Care Cardiovasc Anesth 2010;2:177-90.
12. Coselli JS, LeMaire SA, Preventza O, et al. Outcomes of 3309 thoracoabdominal aortic aneurysm repairs. J Thorac Cardiovasc Surg 2016;151:1323-38.
13. Liang NL, Dake MD, Fischbein MP, et al. Midterm outcomes of endovascular repair of aortic arch aneurysms with the gore thoracic branch endoprosthesis. Eur J Vasc Endovasc Surg 2022;64:639-45.
14. Matsumura JS, Cambria RP, Dake MD, Moore RD, Svensson LG, Snyder S. International controlled clinical trial of thoracic endovascular aneurysm repair with the Zenith TX2 endovascular graft: 1-year results. J Vasc Surg 2008;47:247-57; discussion 257.
15. Motta F, Crowner JR, Kalbaugh CA, et al. Outcomes and complications after fenestrated-branched endovascular aortic repair. J Vasc Surg 2019;70:15-22.
16. Aucoin VJ, Motyl CM, Novak Z, et al. Predictors and outcomes of spinal cord injury following complex branched/fenestrated endovascular aortic repair in the US aortic research consortium. J Vasc Surg 2023;77:1578-87.
17. Tenorio ER, Dias-Neto MF, Lima GBB, Baghbani-Oskouei A, Oderich GS. Lessons learned over 2 decades of fenestrated-branched endovascular aortic repair. Semin Vasc Surg 2022;35:236-44.
18. Wanhainen A, Verzini F, Van Herzeele I, et al. Editor's choice - european society for vascular surgery (ESVS) 2019 clinical practice guidelines on the management of abdominal aorto-iliac artery aneurysms. Eur J Vasc Endovasc Surg 2019;57:8-93.
19. Biglioli P, Roberto M, Cannata A, et al. Upper and lower spinal cord blood supply: the continuity of the anterior spinal artery and the relevance of the lumbar arteries. J Thorac Cardiovasc Surg 2004;127:1188-92.
20. Rojas S, Ortega M, RodríGuez-Baeza A. Variable anatomic configuration of the posterior spinal arteries in humans. Clin Anat 2018;31:1137-43.
21. Colman MW, Hornicek FJ, Schwab JH. Spinal cord blood supply and its surgical implications. J Am Acad Orthop Surg 2015;23:581-91.
22. Hasan S, Arain A. Neuroanatomy, spinal cord arteries. Treasure Island, FL: StatPearls Publishing; 2023.
23. Melissano G, Civilini E, Bertoglio L, Calliari F, Campos Moraes Amato A, Chiesa R. Angio-CT imaging of the spinal cord vascularisation: a pictorial essay. Eur J Vasc Endovasc Surg 2010;39:436-40.
25. Heidemann F, Tsilimparis N, Rohlffs F, et al. Staged procedures for prevention of spinal cord ischemia in endovascular aortic surgery. Gefasschirurgie 2018;23:39-45.
26. Hiratzka LF, Bakris GL, Beckman JA, et al. 2010 ACCF/AHA/AATS/ACR/ASA/SCA/SCAI/SIR/STS/SVM guidelines for the diagnosis and management of patients with thoracic aortic disease: a report of the American College of Cardiology Foundation/American Heart Association Task Force on Practice Guidelines, American Association for Thoracic Surgery, American College of Radiology, American Stroke Association, Society of Cardiovascular Anesthesiologists, Society for Cardiovascular Angiography and Interventions, Society of Interventional Radiology, Society of Thoracic Surgeons, and Society for Vascular Medicine. Circulation 2010;121:e266-369.
27. Coselli JS, LeMaire SA, Miller CC 3rd, et al. Mortality and paraplegia after thoracoabdominal aortic aneurysm repair: a risk factor analysis. Ann Thorac Surg 2000;69:409-14.
28. Agee JM, Flanagan T, Blackbourne LH, Kron IL, Tribble CG. Reducing postischemic paraplegia using conjugated superoxide dismutase. Ann Thorac Surg 1991;51:911-4.
29. Granke K, Hollier LH, Zdrahal P, Moore W. Longitudinal study of cerebral spinal fluid drainage in polyethylene glycol-conjugated superoxide dismutase in paraplegia associated with thoracic aortic cross-clamping. J Vasc Surg 1991;13:615-21.
30. Lintott P, Hafez HM, Stansby G. Spinal cord complications of thoracoabdominal aneurysm surgery. Br J Surg 1998;85:5-15.
31. Fedorow CA, Moon MC, Mutch WA, Grocott HP. Lumbar cerebrospinal fluid drainage for thoracoabdominal aortic surgery: rationale and practical considerations for management. Anesth Analg 2010;111:46-58.
32. Feezor RJ, Lee WA. Strategies for detection and prevention of spinal cord ischemia during TEVAR. Semin Vasc Surg 2009;22:187-92.
33. Xue L, Luo S, Ding H, et al. Risk of spinal cord ischemia after thoracic endovascular aortic repair. J Thorac Dis 2018;10:6088-96.
34. Koo CH, Ryu JH, Hwang JY, Kim JH, Shin HJ, Han SH. Profound hypotension before aortic clamping can exacerbate spinal cord ischemic injury after aortic surgery in rats. J Clin Med 2020;9:3395.
35. Conrad MF, Crawford RS, Davison JK, Cambria RP. Thoracoabdominal aneurysm repair: a 20-year perspective. Ann Thorac Surg 2007;83:S856-61.
36. Kemp CM, Feng Z, Aftab M, Reece TB. Preventing spinal cord injury following thoracoabdominal aortic aneurysm repair: the battle to eliminate paraplegia. JTCVS Tech 2021;8:11-5.
37. Banno H, Kawai Y, Sato T, et al. Low-density vulnerable thrombus/plaque volume on preoperative computed tomography predicts for spinal cord ischemia after endovascular repair for thoracic aortic aneurysm. J Vasc Surg 2021;73:1557-65.E1.
38. Tanaka H, Minatoya K, Matsuda H, et al. Embolism is emerging as a major cause of spinal cord injury after descending and thoracoabdominal aortic repair with a contemporary approach: magnetic resonance findings of spinal cord injury. Interact Cardiovasc Thorac Surg 2014;19:205-10.
39. Isoda S, Izubuchi R, Kawashima J, Yamazaki I, Yano Y, Masuda M. De-airing an open stent graft to potentially reduce spinal cord injury. Gen Thorac Cardiovasc Surg 2019;67:566-8.
40. Ullery BW, Wang GJ, Low D, Cheung AT. Neurological complications of thoracic endovascular aortic repair. Semin Cardiothorac Vasc Anesth 2011;15:123-40.
41. Alizadegan S, Lee CJ. Delayed presentation of spinal cord ischemia after endovascular repair of a thoracoabdominal aneurysm. J Vasc Surg 2015;62:835.
42. Furlan JC, Fehlings MG, Shannon P, Norenberg MD, Krassioukov AV. Descending vasomotor pathways in humans: correlation between axonal preservation and cardiovascular dysfunction after spinal cord injury. J Neurotrauma 2003;20:1351-63.
43. Tsantili AR, Karampelias V, Samolis A, et al. Anatomical variations of human vertebral and basilar arteries: a current review of the literature. Morphologie 2023;107:169-75.
44. Gomes VC, Silvestre GC, Queiroz A, Marques MA, Leão PP, da Silva ES. Biomechanical analysis of cadaveric thoracic aorta zones: the isthmus is the weakest region. Ann Vasc Surg 2021;77:263-73.
45. Rommens KL, Estrera AL. Contemporary management of aortic arch aneurysm. Semin Thorac Cardiovasc Surg 2019;31:697-702.
46. Rehman SM, Vecht JA, Perera R, et al. How to manage the left subclavian artery during endovascular stenting for thoracic aortic dissection? An assessment of the evidence. Ann Vasc Surg 2010;24:956-65.
47. Buth J, Harris PL, Hobo R, et al. Neurologic complications associated with endovascular repair of thoracic aortic pathology: incidence and risk factors. a study from the European collaborators on stent/graft techniques for aortic aneurysm repair (EUROSTAR) registry. J Vasc Surg 2007;46:1103-11.E2.
48. Choo SJ. Commentary: different routes, same destination? J Thorac Cardiovasc Surg 2020;159:1231-2.
49. Dake MD, Brinkman WT, Han SM, et al. Outcomes of endovascular repair of aortic aneurysms with the GORE thoracic branch endoprosthesis for left subclavian artery preservation. J Vasc Surg 2022;76:1141-9.E3.
50. Maldonado TS, Dexter D, Rockman CB, et al. Left subclavian artery coverage during thoracic endovascular aortic aneurysm repair does not mandate revascularization. J Vasc Surg 2013;57:116-24.
51. Riesenman PJ, Farber MA, Mendes RR, Marston WA, Fulton JJ, Keagy BA. Coverage of the left subclavian artery during thoracic endovascular aortic repair. J Vasc Surg 2007;45:90-4.
52. Matsumura JS, Rizvi AZ. Left subclavian artery revascularization: society for vascular surgery practice guidelines. J Vasc Surg 2010;52:65S-70S.
53. Aydin A. Mechanisms and prevention of anterior spinal artery syndrome following abdominal aortic surgery. Angiol Sosud Khir 2015;21:155-64.
54. Picone AL, Green RM, Ricotta JR, May AG, Deweese JA. Spinal cord ischemia following operations on the abdominal aorta. J Vasc Surg 1986;3:94-103.
55. Eagleton MJ, Shah S, Petkosevek D, Mastracci TM, Greenberg RK. Hypogastric and subclavian artery patency affects onset and recovery of spinal cord ischemia associated with aortic endografting. J Vasc Surg 2014;59:89-94.
56. Giosdekos A, Antonopoulos CN, Sfyroeras GS, et al. The use of iliac branch devices for preservation of flow in internal iliac artery during endovascular aortic aneurysm repair. J Vasc Surg 2020;71:2133-44.
57. Simonte G, Parlani G, Farchioni L, et al. Lesson learned with the use of iliac branch devices: single centre 10 year experience in 157 consecutive procedures. Eur J Vasc Endovasc Surg 2017;54:95-103.
58. Schneider DB, Matsumura JS, Lee JT, Peterson BG, Chaer RA, Oderich GS. Five-year outcomes from a prospective, multicenter study of endovascular repair of iliac artery aneurysms using an iliac branch device. J Vasc Surg 2023;77:122-8.
59. Feezor RJ, Martin TD, Hess PJ Jr, et al. Extent of aortic coverage and incidence of spinal cord ischemia after thoracic endovascular aneurysm repair. Ann Thorac Surg 2008;86:1809-14; discussion 1814.
60. Flores J, Kunihara T, Shiiya N, Yoshimoto K, Matsuzaki K, Yasuda K. Extensive deployment of the stented elephant trunk is associated with an increased risk of spinal cord injury. J Thorac Cardiovasc Surg 2006;131:336-42.
61. Czerny M, Eggebrecht H, Sodeck G, et al. Mechanisms of symptomatic spinal cord ischemia after TEVAR: insights from the European registry of endovascular aortic repair complications (EuREC). J Endovasc Ther 2012;19:37-43.
62. Afifi RO, Sandhu HK, Zaidi ST, et al. Intercostal artery management in thoracoabdominal aortic surgery: to reattach or not to reattach? J Thorac Cardiovasc Surg 2018;155:1372-8.e1.
63. Jacobs MJ, de Mol BA, Elenbaas T, et al. Spinal cord blood supply in patients with thoracoabdominal aortic aneurysms. J Vasc Surg 2002;35:30-7.
64. Marturano F, Nisi F, Giustiniano E, Benedetto F, Piccioni F, Ripani U. Prevention of spinal cord injury during thoracoabdominal aortic aneurysms repair: what the anaesthesiologist should know. J Pers Med 2022;12:1629.
65. Etz CD, Zoli S, Mueller CS, et al. Staged repair significantly reduces paraplegia rate after extensive thoracoabdominal aortic aneurysm repair. J Thorac Cardiovasc Surg 2010;139:1464-72.
66. O'Callaghan A, Mastracci TM, Eagleton MJ. Staged endovascular repair of thoracoabdominal aortic aneurysms limits incidence and severity of spinal cord ischemia. J Vasc Surg 2015;61:347-54.E1.
67. Dias-Neto M, Tenorio ER, Huang Y, et al. Comparison of single- and multistage strategies during fenestrated-branched endovascular aortic repair of thoracoabdominal aortic aneurysms. J Vasc Surg 2023;77:1588-97.E4.
68. Geisbüsch S, Stefanovic A, Koruth JS, et al. Endovascular coil embolization of segmental arteries prevents paraplegia after subsequent thoracoabdominal aneurysm repair: an experimental model. J Thorac Cardiovasc Surg 2014;147:220-6.
69. Etz CD, Debus ES, Mohr FW, Kölbel T. First-in-man endovascular preconditioning of the paraspinal collateral network by segmental artery coil embolization to prevent ischemic spinal cord injury. J Thorac Cardiovasc Surg 2015;149:1074-9.
70. Branzan D, Etz Christian D, Moche M, et al. Ischaemic preconditioning of the spinal cord to prevent spinal cord ischaemia during endovascular repair of thoracoabdominal aortic aneurysm: first clinical experience. EuroIntervention 2018;14:828-35.
71. Addas JAK, Mafeld S, Mahmood DN, et al. Minimally invasive segmental artery coil embolization (MISACE) prior to endovascular thoracoabdominal aortic aneurysm repair. Cardiovasc Intervent Radiol 2022;45:1462-9.
72. Petroff D, Czerny M, Kölbel T, et al. Paraplegia prevention in aortic aneurysm repair by thoracoabdominal staging with 'minimally invasive staged segmental artery coil embolisation’ (MIS2ACE): trial protocol for a randomised controlled multicentre trial. BMJ Open 2019;9:e025488.
73. Coselli JS, LeMaire SA, Köksoy C, Schmittling ZC, Curling PE. Cerebrospinal fluid drainage reduces paraplegia after thoracoabdominal aortic aneurysm repair: results of a randomized clinical trial. J Vasc Surg 2002;35:631-9.
74. Suarez-Pierre A, Zhou X, Gonzalez JE, et al. Association of preoperative spinal drain placement with spinal cord ischemia among patients undergoing thoracic and thoracoabdominal endovascular aortic repair. J Vasc Surg 2019;70:393-403.
75. Aucoin VJ, Eagleton MJ, Farber MA, et al. Spinal cord protection practices used during endovascular repair of complex aortic aneurysms by the U.S. aortic research consortium. J Vasc Surg 2021;73:323-30.
76. Parodi FE, Schanzer A, Oderich GS, et al. The development and potential implications of the US Fenestrated and Branched Aortic Research Consortium. Semin Vasc Surg 2022;35:380-4.
77. Kärkkäinen JM, Cirillo-Penn NC, Sen I, et al. Cerebrospinal fluid drainage complications during first stage and completion fenestrated-branched endovascular aortic repair. J Vasc Surg 2020;71:1109-18.E2.
78. Rong LQ, Kamel MK, Rahouma M, et al. Cerebrospinal-fluid drain-related complications in patients undergoing open and endovascular repairs of thoracic and thoraco-abdominal aortic pathologies: a systematic review and meta-analysis. Br J Anaesth 2018;120:904-13.
79. Kakinohana M. What should we do against delayed onset paraplegia following TEVAR? J Anesth 2014;28:1-3.
80. Awad H, Ramadan ME, El Sayed HF, Tolpin DA, Tili E, Collard CD. Spinal cord injury after thoracic endovascular aortic aneurysm repair. Can J Anaesth 2017;64:1218-35.
81. Rinaldi E, Loschi D, Favia N, Santoro A, Chiesa R, Melissano G. Spinal cord ischemia in open and endovascular aortic repair. Aorta 2022;10:194-200.
82. Werndle MC, Saadoun S, Phang I, et al. Monitoring of spinal cord perfusion pressure in acute spinal cord injury: initial findings of the injured spinal cord pressure evaluation study. Crit Care Med 2014;42:646-55.
83. Behzadi F, Simon JE, Zielke TJ, et al. Risk factors associated with spinal cord ischemia during aortic aneurysm repair. Ann Vasc Surg 2023;91:36-49.
84. Tenorio ER, Ribeiro MS, Banga PV, et al. Prospective assessment of a protocol using neuromonitoring, early limb reperfusion, and selective temporary aneurysm sac perfusion to prevent spinal cord injury during fenestrated-branched endovascular aortic repair. Ann Surg 2022;276:e1028-34.
85. Tabayashi K, Motoyoshi N, Akimoto H, et al. Epidural cooling for regional spinal cord hypothermia during most or all of descending thoracic or thoracoabdominal aneurysm repair. Acta Chir Belg 2002;102:224-9.
86. Bobadilla JL, Wynn M, Tefera G, Acher CW. Low incidence of paraplegia after thoracic endovascular aneurysm repair with proactive spinal cord protective protocols. J Vasc Surg 2013;57:1537-42.
87. Donato T, Shapira Y, Artru A, Powers K. Effect of mannitol on cerebrospinal fluid dynamics and brain tissue edema. Anesth Analg 1994;78:58-66.
88. Pascarella A, Manzo L, Bono F. Effect of mannitol bolus administration on cerebrospinal fluid pressure in patients with idiopathic intracranial hypertension: a pilot study. J Neurol 2022;269:6158-64.
89. Kunihara T, Matsuzaki K, Shiiya N, Saijo Y, Yasuda K. Naloxone lowers cerebrospinal fluid levels of excitatory amino acids after thoracoabdominal aortic surgery. J Vasc Surg 2004;40:681-90.
90. Hiramoto JS, Hoffman M, Gasper W, Reilly L, Chuter T. Strict control of blood glucose with an intravenous insulin infusion decreases the risk of post-operative lower extremity weakness after complex endovascular aortic aneurysm repair. Eur J Vasc Endovasc Surg 2019;58:848-53.
91. Hall ED. Neuroprotective actions of glucocorticoid and nonglucocorticoid steroids in acute neuronal injury. Cell Mol Neurobiol 1993;13:415-32.
92. Laschinger JC, Cunningham JN Jr, Cooper MM, Krieger K, Nathan IM, Spencer FC. Prevention of ischemic spinal cord injury following aortic cross-clamping: use of corticosteroids. Ann Thorac Surg 1984;38:500-7.
93. Acher C, Acher CW, Marks E, Wynn M. Intraoperative neuroprotective interventions prevent spinal cord ischemia and injury in thoracic endovascular aortic repair. J Vasc Surg 2016;63:1458-65.
94. Pasqualucci A, Al-Sibaie A, Vaidyan KPT, et al. Epidural corticosteroids, lumbar spinal drainage, and selective hemodynamic control for the prevention of spinal cord ischemia in thoracoabdominal endovascular aortic repair: a new clinical protocol. Adv Ther 2020;37:272-87.
95. Estrera AL, Sheinbaum R, Miller CC 3rd, Harrison R, Safi HJ. Neuromonitor-guided repair of thoracoabdominal aortic aneurysms. J Thorac Cardiovasc Surg 2010;140:S131-5.
96. Sloan TB, Edmonds HL Jr, Koht A. Intraoperative electrophysiologic monitoring in aortic surgery. J Cardiothorac Vasc Anesth 2013;27:1364-73.
97. Kolesár D, Kolesárová M, Pavel J, Dávidová A, Marsala J, Lukácová N. Region-specific sensitivity of the spinal cord to ischemia/reperfusion: the dynamic of changes in catalytic NOS activity. J Physiol Sci 2009;59:97-103.
98. Macdonald DB, Dong CC. Spinal cord monitoring during descending aortic procedures. In: Intraoperative monitoring of neural function. Amsterdam, The Netherlands: Elsevier; 2008. pp. 815-28.
99. Bianchi F, Cursi M, Caravati H, et al. Intraoperative neurophysiologic monitoring in thoracoabdominal aortic aneurysm surgery can provide real-time feedback for strategic decision making. Neurophysiol Clin 2022;52:232-41.
100. Haghighi SS, Sirintrapun SJ, Johnson JC, Keller BP, Oro JJ. Suppression of spinal and cortical somatosensory evoked potentials by desflurane anesthesia. J Neurosurg Anesthesiol 1996;8:148-53.
101. Boezeman RP, van Dongen EP, Morshuis WJ, et al. Spinal near-infrared spectroscopy measurements during and after thoracoabdominal aortic aneurysm repair: a pilot study. Ann Thorac Surg 2015;99:1267-74.
102. Etz CD, Kari FA, Mueller CS, et al. The collateral network concept: a reassessment of the anatomy of spinal cord perfusion. J Thorac Cardiovasc Surg 2011;141:1020-8.
103. Vanpeteghem CM, Van de Moortel LMM, De Hert SG, Moerman AT. Assessment of spinal cord ischemia with near-infrared spectroscopy: myth or reality? J Cardiothorac Vasc Anesth 2020;34:791-6.
104. Kasprzak PM, Gallis K, Cucuruz B, Pfister K, Janotta M, Kopp R. Editor’s choice-temporary aneurysm sac perfusion as an adjunct for prevention of spinal cord ischemia after branched endovascular repair of thoracoabdominal aneurysms. Eur J Vasc Endovasc Surg 2014;48:258-65.
105. Harrison SC, Agu O, Harris PL, Ivancev K. Elective sac perfusion to reduce the risk of neurologic events following endovascular repair of thoracoabdominal aneurysms. J Vasc Surg 2012;55:1202-5.
106. Maurel B, Delclaux N, Sobocinski J, et al. The impact of early pelvic and lower limb reperfusion and attentive peri-operative management on the incidence of spinal cord ischemia during thoracoabdominal aortic aneurysm endovascular repair. Eur J Vasc Endovasc Surg 2015;49:248-54.
107. Ito A, Ito T, Ushijima K. Intraoperative spinal cord ischemia during thoracic endovascular aortic repair under epidural anesthesia: 4AP6-9. Eur J Anaesthesiol 2014;31:70.
108. Tiesenhausen K, Amann W, Koch G, Hausegger KA, Oberwalder P, Rigler B. Cerebrospinal fluid drainage to reverse paraplegia after endovascular thoracic aortic aneurysm repair. J Endovasc Ther 2000;7:132-5.
109. Weissler EH, Voigt SL, Raman V, et al. Permissive hypertension and collateral revascularization may allow avoidance of cerebrospinal fluid drainage in thoracic endovascular aortic repair. Ann Thorac Surg 2020;110:1469-74.
Cite This Article
Export citation file: BibTeX | RIS
OAE Style
Gomes VC, Farber MA, Parodi FE. Strategies for prevention of spinal cord ischemia in the management of thoracic and thoracoabdominal aneurysms. Vessel Plus 2023;7:24. http://dx.doi.org/10.20517/2574-1209.2023.60
AMA Style
Gomes VC, Farber MA, Parodi FE. Strategies for prevention of spinal cord ischemia in the management of thoracic and thoracoabdominal aneurysms. Vessel Plus. 2023; 7: 24. http://dx.doi.org/10.20517/2574-1209.2023.60
Chicago/Turabian Style
Gomes, Vivian Carla, Mark A. Farber, F. Ezequiel Parodi. 2023. "Strategies for prevention of spinal cord ischemia in the management of thoracic and thoracoabdominal aneurysms" Vessel Plus. 7: 24. http://dx.doi.org/10.20517/2574-1209.2023.60
ACS Style
Gomes, VC.; Farber MA.; Parodi FE. Strategies for prevention of spinal cord ischemia in the management of thoracic and thoracoabdominal aneurysms. Vessel Plus. 2023, 7, 24. http://dx.doi.org/10.20517/2574-1209.2023.60
About This Article
Special Issue
Copyright
Data & Comments
Data
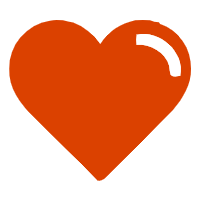

Comments
Comments must be written in English. Spam, offensive content, impersonation, and private information will not be permitted. If any comment is reported and identified as inappropriate content by OAE staff, the comment will be removed without notice. If you have any queries or need any help, please contact us at support@oaepublish.com.