Current state of endovascular repair of thoracoabdominal aortic aneurysms
Abstract
Thoracoabdominal aortic aneurysm (TAAA) is a severe and complex condition with multifactorial etiology that can lead to life-threatening complications. Its treatment is genuinely complex irrespective of the chosen technique, open or endovascular repair. Fenestrated-branched endovascular aneurysm repair (F/B-EVAR) has been increasingly accepted in patients with suitable anatomy, resulting in outcomes compared to or superior to open repair. The selection of patients, judicious surgical planning, and device selection are paramount to achieving successful treatment. The field of TAAA repair is continuously evolving with ongoing research and the development of new techniques and devices to further improve patient outcomes. This paper aims to present a review of endovascular TAAA treatment, summarizing anatomical and clinical features relevant to technical performance and treatment indications.
Keywords
INTRODUCTION
The endovascular treatment of thoracoabdominal aortic aneurysms (TAAAs) continues to evolve over time with novel techniques and devices, expanding the pool of patients eligible for this approach[1]. To successfully repair complex TAAAs, one should take into consideration the anatomy of the patient, clinical characteristics, and the anatomic suitability of each device[2]. Fenestrated and branched grafts have had a crucial role in improving outcomes, allowing treatment for high-risk patients who would not tolerate more invasive procedures[3]. This review brings a comprehensive review of the endovascular treatment of TAAA, presenting a brief historical perspective in addition to anatomical and clinical features relevant to technical success and device selection.
HISTORIC PERSPECTIVE
TAAA repair was first performed by Michael E. DeBakey at the Baylor College of Medicine, Methodist Hospital, in 1953[4]. In the subsequent decades, E. Stanley Crawford was responsible for refining the technique by developing many surgical concepts and a classification system that carries his name, all of which remain relevant today[5,6] [Figure 1].
Figure 1. Classification of thoracoabdominal aortic aneurysm (TAAA) extent based on Crawford. In Extent I the repair comprises most of the descending thoracic and upper abdominal aorta; Extent II involves most of the descending thoracic aorta and most or all of the abdominal aorta; Extent III involves the distal descending thoracic aorta and varying segments of abdominal aorta; and Extent IV involves most or all of the abdominal aorta including the segment from which the visceral vessels arise. (Reproduced by permission of Mayo Foundation for Medical Education and Research. All rights reserved).
The endovascular approach to treat thoracic aortic aneurysms was initially demonstrated by Dake et al. in 1994. They presented the first successful deployment of a stent graft as a thoracic endovascular aortic repair (TEVAR)[7]. In 1999, two case series showed successful endovascular treatments of complicated acute type B dissections with endograft coverage of the proximal entry tear[8,9]. Ultimately, in 2005, the United States Food and Drug Administration (US FDA) approved the first endograft device for human implantation following the completion of the Gore TAG pivotal trial[10].
In 2001, Chuter et al. presented a modular stent-graft system with small branches to pursue the endovascular treatment of TAAAs with preservation of side branches perfusion, offering a less invasive approach[11]. Even though open surgical repair has been considered the preferred approach providing definitive and reliable repair for TAAA, endovascular repair has evolved consistently, presenting a variety of techniques and devices to achieve the treatment of many different aortic pathologies that lead to aneurysm degeneration[10,12-14]. Currently, patient-specific custom-made devices with fenestrations and branches have been developed to match with the anatomy of a specific patient, while standardized off-the-shelf branched devices provide an immediately available solution suitable to common anatomies[15-20] [Figure 2].
Figure 2. Illustration representing the multibranched stent-graft system presented by Chuter et al. in 2001 (A)[11], and the evolution of the manufactured devices (B and C) until the currently standardized off-the-shelf endograft (D). (Reproduced by permissionof Mayo Foundation for Medical Education and Research. All rights reserved).
PATIENT SELECTION
Clinical and anatomical factors
Generally, TAAAs are initially asymptomatic and the disease’s natural history is progression with an increase in diameter, followed by rupture or dissection[21]. In addition, embolization of thrombus associated with the aneurysm wall, or of atheromatous debris, may cause malperfusion syndrome, including mesenteric ischemia, renal ischemia, or peripheral limb ischemia. Erosion of the aneurysm into abutting structures may also occur[22]. Aortic dissection can also further degenerate into aortic aneurysms, and post-dissection aneurysms usually comprise the thoracoabdominal aorta, thereby requiring thoracoabdominal repair. A significant number of patients with acute type B dissections will develop chronic post-dissection aneurysms and need definitive treatment at some stage, either open or with fenestrated/branched stent grafts. Key characteristics in this event are the narrow true lumen of the aorta and visceral branches arising from the false lumen, which applies particularly to either of the renal arteries. In these circumstances, several techniques have been used to cross the thick chronic dissection flap, such as wires with tips that can be stiffened, the back of a wire, a wire with the support of a guiding sheath, or even a needle to perforate the dissection flap[23].
The diameter of the aorta in the thoracoabdominal segment is considered the strongest predictor of adverse events, such as rupture, dissection, and mortality in asymptomatic patients[24]. Elective repair is typically indicated for aortic diameter greater than 6 cm as the limit that justifies surgical risk compared with rupture risk. Aneurysms with a diameter between 5 and 6 cm have a 6.5% annual risk of rupture, dissection, or death. Those greater than 6 cm in diameter have an annual risk as high as 14.1%[25]. The vast majority (93%) of ruptures occur with diameters over 5 cm[21].
Some guidelines suggest repair for aneurysms with a diameter threshold of 5.5 cm, saccular aneurysms larger than 2 cm, or with a total aortic diameter greater than 5 cm[26]. Symptomatic aneurysms or those with rapid expansion (confirmed increase in diameter of ≥ 0.5 cm/y) should also be considered for repair regardless of size or anatomical extent owing to higher risk for rupture. The recommendation for patients with connective tissue disorder is correction at smaller sizes, usually between 4.5 and 5.5 cm[22,27].
The choice of treatment modality should consider the expertise and experience of physicians, anatomy of the patient, and surgical risk assessment. Although the endovascular approach was initially considered only for the elderly or patients with increased surgical risk, its indications have extended to include younger and lower-risk patients with favorable anatomy[28,29]. Older age, poor cardiopulmonary reserve, and multiple comorbidities are features that may favor an endovascular approach, although a comprehensive evaluation should be performed, and patients with exceedingly low life expectancy should not be considered for repair[28].
Open surgical repair is preferred in patients with connective tissue disorders owing to the potential of progressive aortic dilation, fragile landing zones, ultimately leading to low durability of the procedure. Nevertheless, with the recent advent of “off-the-shelf” endografts, TAAA presenting urgently or emergently can now be considered for endovascular treatment[30,31].
DEVICE CHARACTERISTICS
Patient-specific and off-the-shelf stent-grafts
Currently, F/B-EVAR technique includes the use of either patient-specific or off-the-shelf stent grafts[32]. The Zenith Fenestrated AAA Endovascular Graft (ZFEN) was the first patient-specific fenestrated manufactured device to be commercially approved in the United States in April 2012. However, its design accepts the maximum incorporation of three target vessels, resulting in limited use to patients with short neck infrarenal or juxtarenal abdominal aortic aneurysms, excluding those with pararenal aneurysms (PRA) and TAAAs[33]. The use of fenestrated and branched endografts with four or more vessels has been restricted in the United States to clinical trials or institutions with physician-sponsored investigational device exemption (PS-IDE) protocols for patient-specific devices. Moreover, the manufacturing process of these endografts is time-consuming, taking 4-6 weeks, limiting their use in urgent or emergent situations[34]. In this setting, physician-modified endografts (PMEGs) emerge as an option to overcome these obstacles using off-the-shelf endografts which are modified by the surgeon to create fenestrations and directional branches in a sterile manner before implantation to match the anatomy of the patient and clinical needs. One should be aware of the critical importance of the modification planning and technique execution that rely entirely on the surgeon.
Outcomes of PMEGs in comparison with patient-specific manufactured devices (PSMD) have demonstrated it as an effective and safe endovascular option. Data of 316 consecutive treated patients were analyzed, 145 treated with PMEGs (84 PRAs, 26 extent IV, and 35 extent I-III TAAAs), and 171 with PSMD (88 PRAs, 42 extent IV, and 41 extent I-III TAAAs)[32]. Aneurysms repaired with PMEGs were significantly larger, and patients had more chronic pulmonary and kidney disease, and higher comorbidity severity scores. Technical success was high in both groups, slightly lower for PMEGs (98% vs. 99.5%). Early
An important advantage of a patient-specific stent graft is its versatility of design, allowing it to precisely address anatomic differences, such as number of vessels to incorporate, its position, direction, and selection of fenestrations or branches. Single- (10 mm), double- (20 mm), or triple- (30 mm) wide scallops may be manufactured for artery incorporation (albeit not for TAAA) or for catheterization of the device lumen and directional branches can be internal, external, downgoing or upgoing. The graft diameter can be planned with different tapers to better accommodate aortic diameters, and lower profile devices have emerged with smaller delivery systems, such as 20F or even 18F, thus avoiding the use of iliac conduits and decreasing risks of vascular complications[35].
Currently, the Gore Excluder Thoracoabdominal Multi-Branched Endoprosthesis (TAMBE, W.L. Gore & Associates Inc, Flagstaff, AZ) and the Cook Zenith t-Branch graft (Cook Medical, Bloomington, IN, USA) are off-the-shelf alternatives with four downgoing branches; The TAMBE device is currently being investigated in a pivotal trial at selected US centers and likely will be commercially available in the near future for anatomically suitable patients with thoracoabdominal pathology[34,36,37] [Table 1]. Experience with the Cook T-Branch is extensive outside of the USA, with encouraging results including in patients with urgent and emergent presentations[38] [Figures 3 and 4].
Figure 3. The Cook Zenith t-Branch graft is an off-thelf model designed with four downgoing directional cuffs for the celiac axis (CA), superior mesenteric artery (SMA), and both renal arteries (A); Three-dimensional reconstruction of a computed tomographic angiography of a patient with a Type III thoracoabdominal aortic aneurysm treated with a T-Branch stent graft with repair extended to the common iliac arteries bilaterally (B). All branches are bridged with self-expanding covered stents (Viabahn or Fluency) and typically reinforced with a bare-metal stent in its entirety (C). (Reproduced by permission of Mayo Foundation for Medical Educationand Research. All rights reserved).
Figure 4. The Gore Thoracoabdominal Branch Endoprosthesis (TAMBE) with four antegrade portals to accommodate downgoing arteries (A). Three-dimensional reconstruction of a computed tomographic angiography of a patient with a Type IV thoracoabdominal aortic aneurysm treated with the TAMBE device (B). The final aspect of the antegrade repair with distal extension to the common iliac arteries with the Gore Excluder platform and bridging to the target visceral vessels with balloon-expandable VBX endoprosthesis (C). (Reproduced by permission of Mayo Foundation for Medical Education and Research. All rights reserved).
Main characteristics of available or on trial endografts used for FB-EVAR based on their IFU
ZFEN | TAMBE | T-branch | |
Availability | Available on market | On trial | On trial |
Type of device | Patient-specific | Off-the-shelf | Off-the-shelf |
Indication | Short neck and Juxta-renal AAA | TAAA | TAAA |
Fenestration configuration | Up to 03 fenestrations and 01 scallop | 4 downward inner branches | 4 downward branches |
Minimal PLZ (mm) | 4 | 20 | 25 |
PLZ diameter (mm) | 19-31 mm | 22-34 mm (if TAMBE alone) 19.5-32 mm (if proximal stent graft) | 24-30 |
Minimal DLZ (mm) | 30 | 10 | - |
DLZ diameter (mm) | Ipsilateral: 9-21 Contralateral: 7-21 | 8-25 | - |
Aortic neck angle | < 45° | ≤ 60° | < 90° |
Delivery system size | 20 or 22 Fr | 22 Fr | 22 Fr |
Proximal graft diameters (mm) | 24, 26, 28, 30, 32, 34, 36 | 31, 37 | 34 |
Distal graft diameter (mm) | - | 20 | 18 |
Ipsilateral distal graft diameters (mm) | 12, 16, 20, 24 | - | - |
Contralateral distal graft diamenters (mm) | 12, 14, 16, 18, 20, 22, 24 | - | - |
Need for upper extremity access | No | Yes | No |
Upper extremity sheath size | - | 12 F | - |
Inner aortic diameter at the level of the visceral vessels’ origin | - | ≥ 20 mm | - |
Selection of branches or fenestrations
To ensure correct diagnosis and planning, accurate imaging of the complete aortic system and its vessels is mandatory, with computed tomography angiography being the preferred method. Images should be input into a software capable of reconstruction using the centerline of flow, enabling accurate measurement of distances, anatomy of target vessels, aortic size, and angulation. The paramount parameter that should be assessed is the proximal landing zone (PLZ) to certify that attachment sites are free from calcification and thrombus in a healthy aortic segment with suitable angulation[2,39]. A proximal landing zone of at least
In order to incorporate target vessels, the most commonly used methods are fenestrations, directional branches, or a combination of both configurations. While each approach has its benefits and drawbacks, fenestrations are typically preferred for smaller aortic diameters or target arteries with cranial or transverse orientations, while directional branches are favored for wider aortic segments or target arteries that are caudally oriented and tortuous[40,41]. Target arteries that present with early bifurcations are an important factor that should be assessed and, depending on the vessel, imposes technical challenges that could be considered a relative or absolute contraindication to the procedure. Renal arteries and accessory renal arteries with a minimal diameter of 4 mm should be incorporated as coverage or embolization of accessory renal vessels represents a potential risk of acute kidney injury (AKI) and compromised long-term renal function[39,42].
A large space between the aortic endoprosthesis and a target artery ostium is associated with an increased risk of target artery endoleak and potential future reinterventions. Chait et al. demonstrated that the incorporation of target arteries with directional branches presented fewer endoleaks and secondary interventions compared to fenestrations with bridging stents when the distance between the endograft and the target artery ostia was greater than 5 mm[40]. This distance was also independently associated with an increased risk of target artery instability. Even though directional branches are advantageous in certain cases, such as larger aortic aneurysms in which directional cuffs are able to bridge the gap distance between the stent-graft and the target vessel origin, they have demonstrated significantly lower primary and secondary patency, particularly for renal artery, requiring parsimony in its use in patients with known chronic kidney disease, solitary kidneys, or highly tortuous renal vessels[40,43,44].
Tenorio et al., after examining 335 renal-mesenteric arteries targeted by directional branches, showed that the renal artery patency following the incorporation by directional branches appears to be negatively impacted compared to the patency of visceral arteries. Loss of primary patency was found to be associated with renal artery targets as an independent predictor[45].
More recently, results from the United States Aortic Research Consortium (US ARC) revealed that reinforced fenestrations are superior to directional branches in terms of primary and secondary target patency rates, particularly for renal artery incorporation. Primary patency of mesenteric arteries targeted by fenestrations did not differ from those incorporated by directional branches; nonetheless, secondary patency was lower when directional branches were used. When it comes to target artery instability, the study evidenced fewer overall events for vessels targeted using reinforced fenestrations compared to directional branches, but reinforced fenestrations were related to higher rates of type IIIC endoleaks[46].
Bridging stents
The selection of an appropriate bridging stent for F/B-EVAR is largely influenced by the design of the main aortic component[39]. Fenestrated designs usually employ a balloon-expandable stent to secure the target vessel in alignment and subsequently flare it to reduce the risk of component separation. On the other hand, directional branches can be paired with either balloon-expandable or self-expanding stents owing to offering adequate length for overlap, providing a sufficient seal to prevent endoleaks or component separation[47]. The balloon-expandable covered stents currently in use in the United States include iCAST (Atrium Medical, Hudson, NH) or VBX (W. L. Gore & Associates, Flagstaff, AZ), and the self-expanding covered stents used are Viabahn (W. L. Gore & Associates, Flagstaff, AZ) or Fluency (Bard Peripheral Vascular, Tempe, AZ), whereas European centers have access to the Bentley Be-Graft and Be-Graft plus (Bentley InnoMed GmbH, Hechingen) with encouraging results[47].
Directional branches incorporated using balloon-expandable stents have demonstrated lower freedom from target artery instability, endoleak, and secondary intervention compared to self-expandable stent and hybrid stent-graft configuration (a proximal balloon-expandable stent with a distal self-expanding stent)[48] [Figure 5].
Figure 5. Illustration representing the configuration of stents for fenestrations (alignment stents) and branches (bridging stents). The flared segment avoids disconnection and migration of the modules, ensuring optimal alignment and stability of the components, furthermore, facilitating catheterization by the guidewire, if needed. (Reproduced bypermission of Mayo Foundation for Medical Education and Research. All rights reserved).
Access vessel
The selection of vessels for access starts with the study of femoral and iliac vessels to assess for occlusive disease, presence of calcification, diameter, and tortuosity. Currently, the development of novel steerable sheaths has allowed the advancement of stents from a total femoral (TF) access, making this approach preferable, providing relative comfort to the patient and technical ease. Upper extremity (UE) arterial access via brachial or axillary artery may be chosen to favor catheterization of target vessels and allow the passage and delivery of a device with a through-and-through wire system, rectifying tortuous segments and reducing the risk of embolic events. In these situations, a thorough assessment of the aortic arch is necessary[39,49].
Access sites for brachial artery exposure typically consist of an incision at the axillary hairline for larger-diameter sheaths (12 Fr) or at the antecubital crease for smaller-diameter sheaths (7 or 8 Fr). Open brachial access has been preferable over percutaneous, once percutaneous brachial access has been related to more complications than femoral access mainly due to the diameter of the vessel, difficult compression, and proximity of the median nerve, with particular concern about brachial sheath hematoma. Access through the UE is required for the majority of preloaded system devices currently in use[50]. Percutaneous access of the axillary artery has been increasingly utilized and shown similar outcomes to those of open axillary access when vessel closure devices are used as an appropriate exit strategy, particularly in experienced hands[51-53].
More recently, with the widespread availability and improved steerable sheath technology, brachial/axillary access has been relegated to unique cases of difficult target vessel anatomy or extreme aortoiliac tortuosity, as total transfemoral access has been favored by most experienced aortic surgeons. The main advantage of using the TF approach for F/B-EVAR is the reduction of cerebral embolic events and stroke; however, decreasing local brachial/axillary complications and expediting the operation with one less access site are also reported advantages. Moreover, radiation exposure and ergonomics are optimized while avoiding UE exposure. A recent review of 1,681 consecutive F/B-EVAR in the US ARC revealed that the use of brachial access was associated with a twofold increase in the risk of cerebrovascular events, compared to the TF approach (2.8% vs. 1.2%; P = 0.036). Therefore, utilizing the TF approach may be a preferable option whenever possible to minimize the risk of cerebral embolic events and stroke[54]. Despite theoretical concerns regarding a potential increased risk of stroke associated with right UE access compared to left UE access, studies have shown no significant difference[55].
Spinal cord drainage
Spinal cord ischemia (SCI) is a devastating complication following repair of TAAAs, regardless of the type of approach utilized, open or endovascular, and is associated with decreased quality of life and overall survival[56]. Several factors have been demonstrated to affect rates of spinal cord ischemia. The single most important is the extent of aortic disease, with the highest rates observed for type II TAAAs[20]. It is well stablished the necessity of prevention, early identification, and treatment of SCI to prevent permanent paraplegia, overall worse results, and decreased rates of survival[57]. Compromised subclavian, vertebral, and hypogastric perfusion are recognized risk factors for SCI after aortic aneurysm repair[58]. Measures to prevent SCI during endovascular TAAA repair focus on maneuvers to optimize spinal cord perfusion, such as the use of spinal cord drainage (SCD), multistaged approach, and adjunctive procedures such as temporary sac perfusion, early limb reperfusion, and augmentation of blood pressure and cellular oxygen delivery[56]. Currently, our institutional practice has changed to a highly careful selection of patients who undergo prophylactic SCD since this ancillary procedure is not harmless. Patients considered for prophylactic drain placement are primarily those with occlusion of multiple large vessel branches that supply the spinal cord, such as the subclavian and bilateral internal iliac arteries, or in cases in which blood pressure augmentation is considered to be risky, such as symptomatic patients or those with a genetically triggered aortic disease.
EARLY MORTALITY AND MAJOR ADVERSE EVENTS
The primary objective of aneurysm repair is to prevent aortic-related deaths while avoiding significant disability. Crucial outcomes include rates of death and disabling events, such as renal function deterioration, SCI, stroke, myocardial infarction, and respiratory failure. Finally, it is important to evaluate the durability of the repair by assessing secondary interventions, as well as target vessel outcomes.
A systematic review of the literature published in 2020 comparing open and endovascular TAAA repair analyzed 71 studies, of which 24 reported outcomes of F/B-EVAR with a total of 2,059 patients and 47 reported results of open TAAA repair encompassing a total of 12,324 patients. The mean age was 72 in the endovascular group and 63 in the open surgery repair group (P < 0.01). Endovascular patients had more coronary artery disease (44% vs. 31%; P < 0.01), COPD (35% vs. 31%; P = 0.08), and diabetes
A study in Canada compared open and endovascular repair of TAAA. The study evaluated death, permanent SCI, permanent dialysis, and stroke in a composite variable termed “thoracoabdominal aortic aneurysm life-altering events” (TALEs). A total of 664 TAAA repairs were included in the study, of which 303 (45.5%) were endovascular and 361 (54.5%) were open surgery. In-hospital outcomes demonstrated that open repair was associated with a higher incidence of TALE (26.1% vs. 17.4%, P = 0.02); however, no difference was found in the long-term outcomes[60] .
In 2019, Motta et al. published their outcomes of 150 patients treated with F/B-EVAR[61]. PRA and paravisceral aneurysms were included in the group of type IV aneurysms based on the frequent necessity of incorporation of all four visceral vessels in either type[61]. The early mortality demonstrated by that study was 2.7%. Major adverse events included respiratory insufficiency in 10 patients (7%), stroke and myocardial infarction in 0.7% each (1/150), and paraplegia in 2.7% (4 patients). AKI was identified in 4.7% of patients (7/150), with two patients requiring temporary dialysis[61].
More recently, Oderich et al. reported the midterm outcomes of a prospective, nonrandomized study with 430 patients who underwent F/BEVARs, of which 297 had TAAAs and 133 had PRA[62]. The 30-day overall mortality was 0.9%; for TAAAs, extent IV was 1.5%, and for extent I to III was 0.6%. The incidence of paraplegia was significantly higher (P < 0.004) in patients with Extent I-III TAAAs (8%) as compared to Extent IV or PRAs (2% each), as expected. Among the 17 patients who developed SCI, 7 of them achieved total or partial recovery and were able to ambulate within one month, whereas 7 patients (4%) treated for Extent I-III TAAAs suffered from permanent paraplegia. Interestingly, 10 patients with prophylactic spinal cord drainage (SCD) had paraplegia. Out of 10 patients with prophylactic SCD, 2 patients experienced paraplegia resulting from drain complications, such as spinal hematoma, which led to a change in practice in our group to the utilization of SCD in selected cases. The mean days of hospitalization were 6.5 ± 6.1 days and were significantly longer (P < 0.001) in patients treated for Extent I-III TAAAs (10 ± 11 days) in comparison to those treated for Extent IV and PRAs (6 ± 5 and 5 ± 5 days), respectively[62].
Other researchers assessed patients who underwent F/B-EVAR between 2008 and 2019 in four Italian university centers. A total of 596 patients were included in the study, and of those, 351 were TAAA. Early outcomes were more ominous than those reported by Oderich and Motta, with 8% of mortality, and a high rate of renal (17%), pulmonary (12%), and cardiac (9%) complications. SCI was identified in 11% of cases, and permanent paraplegia was identified in 5%. Other contemporary reports consistently show in-hospital mortalities for F/B-EVAR in the range of 2% to 5.6% for TAAAs, and more recently, US ARC from nine PS-IDE studies demonstrated an overall mortality of 3% [Table 2][63-65].
Thoracoabdominal F/B-EVAR - Early outcomes
Author | Year | n | Extent | MAE | Death | AKI | Dialysis | Respiratory failure | MI | SCI | Temporary paraplegia | Permanent paraplegia | Any stroke | GI resection | HLOS (days) |
Oikonomou et al.[63] n (%) or mean (± SD) | 2019 | 71 | - | 13 (18,3) | 4 (5.6) | 2 (2.8) | 2 (2.8) | - | - | 11 (15.5) | 9 (12,7) | 2 (2.8) | - | - | 11 (6-48) |
Law et al.[64] n (%) or mean (± SD) | 2019 | 20 | - | - | 1 (5) | 5 (25) | - | 3 (15) | - | 2 (10) | 1 (5) | 1 (5) | - | - | 9 (± 13) |
Motta et al.[61] n (%) or mean (± SD) | 2019 | 150 | - | 39 (26) | 4 (2.7) | 7 (4.7) | 2 (1.3) | 10 (7) | 1 (0.7) | 4 (2,7) | 1 (0.7) | 3 (2) | 1 (0.7) | - | 5.6 (± 5.4) |
Rocha et al.[59] (%) or mean (± SD) | 2020 | 2,059 | - | - | (7.4) | (11.7) | (6.4) | - | - | (13.5) | - | (5.2) | (2.7) | - | 12.8 (7,8-17,7) |
Oderich et al.[62] n (%), (%) or mean | 430 | IV (132) | 30 (23) | (1.5) | 24 (18) | 3 (2) | 4(3) | 6 (5) | 3 (2) | 2 (2) | 1 (1) | 4 (3) | 2 (2) | 6.1 (± 4.6) | |
2021 | I - III (165) | 34 (21) | (0.6) | 18 (11) | 3 (2) | 7 (4) | 1 (1) | 20 (12) | 13 (8) | 7 (4) | 5 (3) | 0 (0) | 9.6 (± 10.7) | ||
PRA (133) | 19 (14) | (0.8) | 14 (11) | 2 (2) | 1 (1) | 11 (3) | 2 (2) | 0 (0) | 2 (2) | 1 (1) | 4 (3) | 4.8 (± 5.4) | |||
Zettervall et al.[65] US ARC n (%) or mean (± SD) | 2022 | 1,681 | I-III (644) IV (535) CAAA (502) | 423 (25) | 46 (3) | 161 (10) | 25 (2) | 56 (3) | 35 (2) | 120 (7) | 81 (5) | 39 (2) | 32 (2) | - | 6.8 (± 9) |
SECONDARY INTERVENTIONS AND LATE OUTCOMES
While recent series have reported favorable outcomes regarding safety with F/B-EVAR, a notable concern remains the high rate of secondary interventions. According to data published by Zettervall et al., after analyzing 1,681 cases from the US ARC, 385 (23%) needed a secondary intervention. The follow-up period had a mean duration of 23 months. Freedom from reintervention rates were reported as 82% and 59% at 1 and 5 years of follow-up, respectively. Endoleaks were the main indication for secondary intervention, accounting for 57% of the secondary interventions[65]. Stenosis and occlusion were also reported to represent 10% of the indications. As secondary interventions, renal stenting was the most frequent procedure (30%), followed by open treatment of access site complications (24%). Conversion to open repair was necessary in six patients and 16 patients had aneurysm rupture demanding repair[65]. Technical success rate for secondary interventions was 94%, and the mortality rate associated with these interventions was less than 1%. The study found that, overall, secondary interventions were associated with improved survival, with a hazard ratio of 0.6 and a 95% confidence interval ranging from 0.5 to 0.7[65].
Similar findings were shared by Oderich et al., who reported 23% of secondary interventions in 430 cases[62]; in that series, freedom from secondary intervention at 1, 3, and 5 years was 81%, 74%, and 64%, respectively. Freedom from target artery instability was reported to be 89% and significantly lower for renal arteries (85%) compared to superior mesenteric arteries (SMA) (91%) and celiac axis (97%), P < 0.001. As previously mentioned, directional branches had lower freedom from target artery instability compared to reinforced fenestrations, with rates of 87% and 91% (P = 0.03), respectively[62]. Target vessel patency followed similar trends, revealing that primary and secondary patency rates were significantly lower for renal arteries compared to SMA and celiac axis. Specifically, the primary patency rate for renal arteries was 89%, while the secondary patency rate was 95%. In contrast, the SMA exhibited a primary patency rate of 97% and a secondary patency rate of 99% (P < 0.001). The celiac axis demonstrated even higher rates, with a primary patency rate of 99% and a secondary patency rate of 99% (P = 0.02). At 5 years, the primary patency of all target vessels was 94%, while the secondary patency was 97%. Reinforced fenestrations had a significantly higher primary patency rate at 5 years compared to directional branches (95% vs. 91%, P = 0.036)[62]. These differences in stability and patency rates of target vessels suggest variations in the long-term effectiveness of vessel repair in different anatomical locations and the influence of incorporation technique.
Outcomes on late mortality were recently published by Chait et al. after analyzing 156 patients treated with PMEGs (89 complex AAAs, 33 type IV TAAAs, and 34 type I to III TAAAs)[66]. After a mean follow-up of 49 ± 38 months, there were 12 aortic-related deaths (7.6%), including nine early deaths (5.7%) from perioperative complications and three late deaths (1.9%) from rupture. At 5 years, patient survival was 41%. Results on open surgery repair were also analyzed by Coselli et al.[19]. Out of 3,309 open repairs included in the study, there were 1,864 late deaths[19]. Estimated survival was 83.5% ± 0.7% at 1 year, 63.6% ± 0.9% at
CONCLUSION
Endovascular treatment of TAAA has evolved widely and gained widespread acceptance due to reduced morbidity and mortality compared to open repair. Formerly reserved to treat high-risk patients, its indications are currently broadened and include young patients with suitable anatomy. Meticulous surgical planning, appropriate patient and device selection, and precise implantation technique are key to achieving optimal outcomes. Finally, technological advancements continue to develop rapidly, favoring better outcomes and expanding indications for this modality of repair.
DECLARATIONS
Authors’ contributions
Study conception and design, analysis and interpretation of data, drafting of manuscript, critical revision, final approval: Rodrigues DVS, Lima GBB, DeMartino RR, Mendes BC
Acquisition of data: Rodrigues DVS, Lima GBB
Availability of data and materials
Not applicable.
Financial support and sponsorship
None.
Conflicts of interest
All authors declared that there are no conflicts of interest.
Ethical approval and consent to participate
Not applicable.
Consent for publication
Not applicable.
Copyright
© The Author(s) 2024.
REFERENCES
1. Nation DA, Wang GJ. TEVAR: endovascular repair of the thoracic aorta. Semin Intervent Rad 2015;32:265-71.
2. Chait J, Mendes BC, DeMartino RR. Anatomic factors to guide patient selection for fenestrated-branched endovascular aortic repair. Semin Vasc Surg 2022;35:259-79.
3. Paajanen P, Kärkkäinen JM, Tenorio ER, Mendes BC, Oderich GS. Effect of patient frailty status on outcomes of fenestrated-branched endovascular aortic repair for complex abdominal and thoracoabdominal aortic aneurysms. J Vasc Surg 2022;76:1170-9.e2.
4. De Bakey ME, Cooley DA. Successful resection of aneurysm of thoracic aorta and replacement by graft. J Am Med Assoc 1953;152:673-6.
5. Crawford ES. Thoraco-abdominal and abdominal aortic aneurysms involving renal, superior mesenteric, celiac arteries. Ann Surg 1974;179:763-72.
6. Crawford ES, Crawford JL, Safi HJ, et al. Thoracoabdominal aortic aneurysms: preoperative and intraoperative factors determining immediate and long-term results of operations in 605 patients. J Vasc Surg 1986;3:389-404.
7. Dake MD, Miller DC, Semba CP, Mitchell RS, Walker PJ, Liddell RP. Transluminal placement of endovascular stent-grafts for the treatment of descending thoracic aortic aneurysms. N Engl J Med 1994;331:1729-34.
8. Dake MD, Kato N, Mitchell RS, et al. Endovascular stent-graft placement for the treatment of acute aortic dissection. N Engl J Med 1999;340:1546-52.
9. Nienaber CA, Fattori R, Lund G, et al. Nonsurgical reconstruction of thoracic aortic dissection by stent-graft placement. N Engl J Med 1999;340:1539-45.
10. Makaroun MS, Dillavou ED, Kee ST, et al. Endovascular treatment of thoracic aortic aneurysms: results of the phase II multicenter trial of the GORE TAG thoracic endoprosthesis. J Vasc Surg 2005;41:1-9.
11. Chuter TA, Gordon RL, Reilly LM, Goodman JD, Messina LM. An endovascular system for thoracoabdominal aortic aneurysm repair. J Endovasc Ther 2001;8:25-33.
12. Riesenman PJ, Reeves JG, Kasirajan K. Endovascular management of a ruptured thoracoabdominal aneurysm-damage control with superior mesenteric artery snorkel and thoracic stent-graft exclusion. Ann Vasc Surg 2011;25:555.e5-9.
13. Marchal J, Verhelst R, Astarci P, Elens M. Endovascular repair of a ruptured thoracoabdominal aortic aneurysm with the sandwich technique: a case report. Vasc Endovascular Surg 2021;55:86-90.
14. Benjelloun A, Henry M, Ghannam A, et al. Endovascular treatment of a tuberculous thoracoabdominal aneurysm with the multilayer stent. J Endovasc Ther 2012;19:115-20.
15. Moulakakis KG, Karaolanis G, Antonopoulos CN, et al. Open repair of thoracoabdominal aortic aneurysms in experienced centers. J Vasc Surg 2018;68:634-45.e12.
16. Cowan JA Jr, Dimick JB, Henke PK, Huber TS, Stanley JC, Upchurch GR Jr. Surgical treatment of intact thoracoabdominal aortic aneurysms in the United States: hospital and surgeon volume-related outcomes. J Vasc Surg 2003;37:1169-74.
17. Cambria RP, Clouse WD, Davison JK, Dunn PF, Corey M, Dorer D. Thoracoabdominal aneurysm repair: results with 337 operations performed over a 15-year interval. Ann Surg 2002;236:471-9.
18. Svensson LG, Crawford ES, Hess KR, Coselli JS, Safi HJ. Experience with 1509 patients undergoing thoracoabdominal aortic operations. J Vasc Surg 1993;17:357-68.
19. Coselli JS, LeMaire SA, Preventza O. Outcomes of 3309 thoracoabdominal aortic aneurysm repairs. J Thorac Cardiovasc Surg 2016;151:1323-37.
20. Greenberg RK, Lu Q, Roselli EE, et al. Contemporary analysis of descending thoracic and thoracoabdominal aneurysm repair: a comparison of endovascular and open techniques. Circulation 2008;118:808-17.
21. Zafar MA, Chen JF, Wu J, et al. Yale Aortic Institute Natural History Investigators. Natural history of descending thoracic and thoracoabdominal aortic aneurysms. J Thorac Cardiovasc Surg 2021;161:498-511.e1.
22. Isselbacher EM, Preventza O, Hamilton Black J 3rd, et al. 2022 ACC/AHA guideline for the diagnosis and management of aortic disease: a report of the American heart association/American college of cardiology joint committee on clinical practice guidelines. Circulation 2022;146:e334-482.
23. Verhoeven EL, Paraskevas KI, Oikonomou K, et al. Fenestrated and branched stent-grafts to treat post-dissection chronic aortic aneurysms after initial treatment in the acute setting. J Endovasc Ther 2012;19:343-9.
24. Ziganshin BA, Elefteriades JA. Surgical management of thoracoabdominal aneurysms. Heart 2014;100:1577-82.
25. Elefteriades JA. Natural history of thoracic aortic aneurysms: indications for surgery, and surgical versus nonsurgical risks. Ann Thorac Surg 2002;74:S1877-80.
26. Svensson LG, Kouchoukos NT, Miller DC, et al. Society of Thoracic Surgeons Endovascular Surgery Task Force. Expert consensus document on the treatment of descending thoracic aortic disease using endovascular stent-grafts. Ann Thorac Surg 2008;85:S1-41.
27. Hiratzka LF, Bakris GL, Beckman JA, et al. 2010 ACCF/AHA/AATS/ACR/ASA/SCA/SCAI/SIR/STS/SVM guidelines for the diagnosis and management of patients with thoracic aortic disease: a report of the American college of cardiology foundation/American heart association task force on practice guidelines, American association for thoracic surgery, American college of radiology, American stroke association, society of cardiovascular anesthesiologists, society for cardiovascular angiography and interventions, society of interventional radiology, society of thoracic surgeons, and society for vascular medicine. Circulation 2010;121:e266-369.
28. Ouzounian M, Tadros RO, Svensson LG, Lyden SP, Oderich GS, Coselli JS. Thoracoabdominal aortic disease and repair: JACC focus seminar, part 3. J Am Coll Cardiol 2022;80:845-56.
29. Rocha RV, Friedrich JO, Elbatarny M, et al. A systematic review and meta-analysis of early outcomes after endovascular versus open repair of thoracoabdominal aortic aneurysms. J Vasc Surg 2018;68:1936-45.e5.
30. Bertoglio L, Cambiaghi T, Ferrer C, et al. Comparison of sacrificed healthy aorta during thoracoabdominal aortic aneurysm repair using off-the-shelf endovascular branched devices and open surgery. J Vasc Surg 2018;67:695-702.
31. Spanos K, Kodolitsch Y, Detter NC, et al. Endovascular treatment of aortic aneurysms and dissections in patients with genetically triggered aortic diseases. Semin Vasc Surg 2022;35:320-33.
32. Oderich GS, Ribeiro MS, Sandri GA, et al. Evolution from physician-modified to company-manufactured fenestrated-branched endografts to treat pararenal and thoracoabdominal aortic aneurysms. J Vasc Surg 2019;70:31-42.e7.
33. Oderich GS, Greenberg RK, Farber M, et al. Zenith Fenestrated Study Investigators. Results of the United States multicenter prospective study evaluating the zenith fenestrated endovascular graft for treatment of juxtarenal abdominal aortic aneurysms. J Vasc Surg 2014;60:1420-8.e1.
34. Pyun AJ, Han SM. Contemporary indications, techniques, and outcomes of physician-modified endografts for the treatment of complex abdominal and thoracoabdominal aortic aneurysms. Semin Vasc Surg 2022;35:364-73.
35. Oderich GS, Ribeiro M, Hofer J, et al. Prospective, nonrandomized study to evaluate endovascular repair of pararenal and thoracoabdominal aortic aneurysms using fenestrated-branched endografts based on supraceliac sealing zones. J Vasc Surg 2017;65:1249-59.e10.
36. Oderich GS, Farber MA, Silveira PG, et al. Technical aspects and 30-day outcomes of the prospective early feasibility study of the GORE EXCLUDER thoracoabdominal branched endoprosthesis (TAMBE) to treat pararenal and extent IV thoracoabdominal aortic aneurysms. J Vasc Surg 2019;70:358-68.e6.
37. Atkins AD, Atkins MD. Branched and fenestrated aortic endovascular grafts. Methodist Debakey Cardiovasc J 2023;19:15-23.
38. Kölbel T, Spanos K, Jama K, et al. Early outcomes of the t-branch off-the-shelf multi-branched stent graft in 542 patients for elective and urgent aortic pathologies: a retrospective observational study. J Vasc Surg 2021;74:1817-24.
39. Zettervall SL, Starnes BW. Planning and sizing of fenestrated/branched stent grafts. Semin Vasc Surg 2022;35:252-8.
40. Chait J, Tenorio ER, Mendes BC, et al. Impact of gap distance between fenestration and aortic wall on target artery instability following fenestrated-branched endovascular aortic repair. J Vasc Surg 2022;76:79-87.e4.
41. Katsargyris A, Marques de Marino P, Verhoeven EL. Graft design and selection of fenestrations vs. branches for renal and mesenteric incorporation in endovascular treatment of pararenal and thoracoabdominal aortic aneurysms. J Cardiovasc Surg 2019;60:35-40.
42. Mendes BC, Oderich GS, Reis de Souza L, et al. Implications of renal artery anatomy for endovascular repair using fenestrated, branched, or parallel stent graft techniques. J Vasc Surg 2016;63:1163-9.e1.
43. Martin-Gonzalez T, Mastracci T, Carrell T, et al. Mid-term outcomes of renal branches versus renal fenestrations for thoraco-abdominal aneurysm repair. Eur J Vasc Endovasc Surg 2016;52:141-8.
44. Sugimoto M, Panuccio G, Bisdas T, Berekoven B, Torsello G, Austermann M. Tortuosity is the significant predictive factor for renal branch occlusion after branched endovascular aortic aneurysm repair. Eur J Vasc Endovasc Surg 2016;51:350-7.
45. Tenorio ER, Kärkkäinen JM, Mendes BC, et al. Outcomes of directional branches using self-expandable or balloon-expandable stent grafts during endovascular repair of thoracoabdominal aortic aneurysms. J Vasc Surg 2020;71:1489-502.e6.
46. Tenorio ER, Schanzer A, Timaran CH, et al. U. S. Fenestrated and Branched Aortic Research Consortium. Mid-term renal and mesenteric artery outcomes during fenestrated and branched endovascular aortic repair for complex abdominal and thoracoabdominal aortic aneurysms in the united states aortic research consortium. Ann Surg 2023;278:e893-902.
47. Kim S, Parodi FE, Farber MA. Choice of optimal bridging stent and methods of visceral vessel incorporation during fenestrated/branched endovascular aortic repair for thoracoabdominal and complex abdominal aortic aneurysms. Semin Vasc Surg 2022;35:280-6.
48. Tenorio ER, Schanzer A, Timaran CH, et al. U. S. Fenestrated and Branched Aortic Research Consortium. Effect of bridging stent graft selection for directional branches on target artery outcomes of fenestrated-branched endovascular aortic repair in the United States Aortic Research Consortium. J Vasc Surg 2023;78:10-28.e3.
49. Lima GBB, Dias-Neto M, Tenorio ER, Baghbani-Oskouei A, Oderich GS. Endovascular repair of complex aortic aneurysms. Adv Surg 2022;56:305-19.
50. Porras-Colon J, Knowles M, Timaran CH. Outcomes and strategies for utilization of brachial access and preloaded systems during
51. Bertoglio L, Mascia D, Cambiaghi T, Kahlberg A, Melissano G, Chiesa R. Percutaneous axillary artery access for fenestrated and branched thoracoabdominal endovascular repair. J Vasc Surg 2018;68:12-23.
52. Agrusa CJ, Connolly PH, Ellozy SH, Schneider DB. Safety and effectiveness of percutaneous axillary artery access for complex aortic interventions. Ann Vasc Surg 2019;61:326-33.
53. Al Adas Z, Uceda D, Mazur A, et al. Safety and learning curve of percutaneous axillary artery access for complex endovascular aortic procedures. J Vasc Surg 2024;79:487-96.
54. Chamseddin KH, Timaran CH, Scott CK, et al. Outcomes of upper extremity versus transfemoral access for fenestrated-branched endovascular aortic repair. J Vasc Surg 2022;75:e41.
55. Mirza AK, Tenorio ER, Marcondes GB, et al. Comparison of cerebral embolic events between right and left upper extremity access during fenestrated/branched endovascular aortic repair. J Endovasc Ther 2021;28:70-7.
56. Motyl CM, Beck AW. Strategies for prevention and treatment of spinal cord ischemia during F/BEVAR. Semin Vasc Surg 2022;35:297-305.
57. Aucoin VJ, Bolaji B, Novak Z, et al. Trends in the use of cerebrospinal drains and outcomes related to spinal cord ischemia after thoracic endovascular aortic repair and complex endovascular aortic repair in the vascular quality initiative database. J Vasc Surg 2021;74:1067-78.
58. Griepp RB, Griepp EB. Spinal cord perfusion and protection during descending thoracic and thoracoabdominal aortic surgery: the collateral network concept. Ann Thorac Surg 2007;83:S865-9.
59. Rocha RV, Lindsay TF, Friedrich JO, et al. Systematic review of contemporary outcomes of endovascular and open thoracoabdominal aortic aneurysm repair. J Vasc Surg 2020;71:1396-412.e12.
60. Rocha RV, Lindsay TF, Austin PC, et al. Outcomes after endovascular versus open thoracoabdominal aortic aneurysm repair: a population-based study. J Thorac Cardiovasc Surg 2021;161:516-27.e6.
61. Motta F, Crowner JR, Kalbaugh CA, et al. Outcomes and complications after fenestrated-branched endovascular aortic repair. J Vasc Surg 2019;70:15-22.
62. Oderich GS, Tenorio ER, Mendes BC, et al. Midterm outcomes of a prospective, nonrandomized study to evaluate endovascular repair of complex aortic aneurysms using fenestrated-branched endografts. Ann Surg 2021;274:491-9.
63. Oikonomou K, Kasprzak P, Katsargyris A, Marques De Marino P, Pfister K, Verhoeven ELG. Mid-term results of fenestrated/branched stent grafting to treat post-dissection thoraco-abdominal aneurysms. Eur J Vasc Endovasc Surg 2019;57:102-9.
64. Law Y, Tsilimparis N, Rohlffs F, et al. Fenestrated or branched endovascular aortic repair for postdissection thoracoabdominal aortic aneurysm. J Vasc Surg 2019;70:404-12.
65. Zettervall SL, Tenorio ER, Schanzer A, et al. U.S. Fenestrated and Branched Aortic Research Consortium. . Secondary interventions after fenestrated/branched aneurysm repairs are common and nondetrimental to long-term survival. J Vasc Surg 2022;75:1530-8.e4.
Cite This Article
Export citation file: BibTeX | RIS
OAE Style
Rodrigues DVS, Lima GBB, DeMartino RR, Mendes BC. Current state of endovascular repair of thoracoabdominal aortic aneurysms. Vessel Plus 2024;8:10. http://dx.doi.org/10.20517/2574-1209.2023.109
AMA Style
Rodrigues DVS, Lima GBB, DeMartino RR, Mendes BC. Current state of endovascular repair of thoracoabdominal aortic aneurysms. Vessel Plus. 2024; 8: 10. http://dx.doi.org/10.20517/2574-1209.2023.109
Chicago/Turabian Style
Rodrigues, Diego V. S., Guilherme B. Barbosa Lima, Randall R. DeMartino, Bernardo C. Mendes. 2024. "Current state of endovascular repair of thoracoabdominal aortic aneurysms" Vessel Plus. 8: 10. http://dx.doi.org/10.20517/2574-1209.2023.109
ACS Style
Rodrigues, DVS.; Lima GBB.; DeMartino RR.; Mendes BC. Current state of endovascular repair of thoracoabdominal aortic aneurysms. Vessel Plus. 2024, 8, 10. http://dx.doi.org/10.20517/2574-1209.2023.109
About This Article
Special Issue
Copyright
Data & Comments
Data
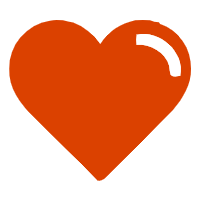

Comments
Comments must be written in English. Spam, offensive content, impersonation, and private information will not be permitted. If any comment is reported and identified as inappropriate content by OAE staff, the comment will be removed without notice. If you have any queries or need any help, please contact us at support@oaepublish.com.