Descending thoracic and thoracoabdominal aortic aneurysm repair using deep hypothermic circulatory arrest
Abstract
Descending thoracic and thoracoabdominal aortic replacement is a complex and high-risk surgery. Deep hypothermic circulatory arrest (DHCA) is a surgical technique that is useful in large distal aortic arch aneurysms or chronic dissections that require fenestration where proximal cross clamping would be difficult. It can also be used as part of a multimodal strategy for end-organ protection. However, DHCA has potential adverse effects on the myocardial, cerebral, pulmonary, and coagulation systems. The use of DHCA is guided by the experience and preferences of the surgical team as well as the technical demands of the proposed surgery.
Keywords
INTRODUCTION
Descending thoracic (DTA) and thoracoabdominal aortic (TAAA) replacement is a complex and high-risk surgery. Extensive anatomic exposure is required; necessitating a thoracotomy or a thoraco-phreno-laparotomy which can lead to pulmonary complications, diaphragmatic dysfunction, as well as compromised wound healing[1]. Organ dysfunction is common and is due to a combination of potential atheroembolic events, ischemic-reperfusion injury, and hemodynamic changes during aortic cross-clamping[2]. Despite multimodal advances in intraoperative and postoperative care, rates of both mortality and morbidity including spinal cord ischemia, cardiac, pulmonary, and neurologic events, respiratory compromise, and renal dysfunction remain significant[3]. Inherent in the conduct of open repair of DTA/TAAA is a period during which there is regional ischemia to the visceral organs; and most of the variability between surgical technique lies in the method selected to provide organ protection[4]. The rate of renovisceral organ dysfunction after DTA and TAAA repair is widely reported in the literature, depending on the clinical and biochemical criteria used to define each, however it ranges from 21%-63%[5]. Other organ systems are affected by periods of ischemia, notably the spinal cord; rates of spinal cord injury, both temporary and permanent, have been reported as high as 20% for open repair of DTA/TAAA, with the caveat that these rates are most affected by the extent of repair[6]. While it is difficult to ascertain the true value due to the heterogeneity of disease extent, indication for surgery, replacement techniques, adjunctive protection strategies, and the potential for improved outcomes from experiences centres, mortality rates typically range from 5 to 33%[7].Pertinent to the discussion on the use of deep hypothermic circulatory arrest in the repair of DTA and TAAAs is the specific considerations of aortic cross-clamping. Fundamentally, the application of an aortic cross-clamp leads to hemodynamic sequelae proximal to the clamp, and ischemic sequelae distal to the clamp, as well as potential clamp-related complications such as vascular trauma, iatrogenic aortic dissection, and atheroembolism. Surgical techniques aimed at organ protection primarily seek to reduce the impact of distal ischemia imposed on the body by the application of an aortic cross-clamp, the most intuitive approach being the continuation of blood perfusion to these organs despite the interruption of native blood flow. This is accomplished by the utilization of either left-heart bypass (LHB) or cardiopulmonary bypass (CPB), employing various cannulation strategies aimed at the maintenance of perfusion distal to the aortic cross-clamp.
The rationale for using DHCA in TAAA repair are heavily calcified and non-clampable distal aortic arch or proximal descending thoracic aorta which include massive aneurysms with distorted anatomy or the need to fenestrate a dissection flap in the aortic arch be facilitated by open endoluminal aortic exposure. To facilitate overcoming these challenges for aortic replacement for DTAs and TAAAs is the use of CPB with DHCA. Defined as the achievement of systemic temperatures between 18-24 degrees Celsius accomplished using cardiopulmonary bypass, it facilitates cerebral, cardiac and organ protection during operations necessitating cessation of systemic circulation.
An assessment of the literature on techniques for DTA/TAAA repair reveals some selection criteria regarding the use of DHCA. In summary, the main identifiable criteria that support the use of DHCA include severe aortic atherosclerosis which would increase embolic risk with clamping, contained arch aneurysm rupture, as well as a porcelain aorta that precludes cross-clamping. Other potential factors may include elevated risk of spinal cord ischemia as well as reduced preoperative renal function, as DHCA may provide superior spinal cord and visceral organ protection than other perfusion adjuncts at higher patient temperatures[8]. Additional factors to consider are if the patient has had prior central aortic procedures performed, as the re-operative tissue may increase risk of injury to adjacent structures such as the esophagus, bronchus, pulmonary arteries, and left recurrent laryngeal nerve[9]. Furthermore, clamp-induced aortic injury, retrograde aortic dissection from clamping, as well as massive, uncontrollable bleeding would necessitate the use of DHCA. Beyond these, there are few clinical or patient factors reported for the selection of DHCA use for these complex repairs. Pre-operatively identified reduced left ventricular ejection fraction, as well as aortic valve insufficiency, may be negative predictive factors for utilizing DHCA. This is due to multiple risks associated with using DHCA for DTA/TAAA repair: inadequate myocardial protection during hypothermic fibrillatory arrest, hypothermic tissue damage and consequent myocardial edema, prolonged CPB times because of cooling and re-warming and left ventricular distention which may be because of aortic valve insufficiency[8]. Various adjuncts can be used to monitor and avoid these complications, including intraoperative pulmonary artery catheter pressure recordings, transesophageal echocardiography, as well as placement of a left inferior pulmonary vein vent which can both augment venous drainage as well as decompressing the left ventricle. However, there is a paucity of patient factors that have been assessed as favorable or prohibitive for DHCA in the repair of DTAs/TAAAs. One such factor may be obesity, which poses both surgical and perfusion-related challenges on the execution of TAAA repair. The severity of obesity in relation to CPB is often reflected in both with the body mass index [BMI] as well as the body surface area [BSA] calculations. Most studies on the safety of various forms of cardiac surgery, including those utilizing DHCA, have identified elevated BMI as an independent risk factor for postoperative complications such as myocardial infarction, sepsis, pulmonary and gastrointestinal sequelae, as well as overall mortality[10]. While there are numerous additional challenges posed by obesity on the conduct of CPB, there are some specific challenges related to cases using DHCA. As higher flow rates are required for larger patients, this necessitates higher venous return, and a much larger venous reservoir may be required to accommodate the larger circulating volume of these patients during the exsanguination phase of DHCA[11]. However, there have been no dedicated analyses performed to date looking at the implications of obesity specifically on the outcomes of DTA/TAAA repair using DHCA. At this point, there can be no recommended cut-offs on the applicability of DHCA to the repair of these complex pathologies, as the more salient technical and clinical factors as detailed above seem to be more impactful.
There are numerous medical adjuncts used in the efforts to improve the safety of performing thoracoabdominal aneurysm repair with DHCA. One such strategy involves the administration of systemic corticosteroids, either intravenously or delivered via the CPB reservoir, to improve neurologic outcomes. There is considerable variation in the literature regarding the timing and dosing of these medications, with some advocating administration at least 6-8 h pre-operatively, to their delivery intraoperatively[12]. Nevertheless, the administration of adjunctive medication, be it corticosteroids or otherwise, is a practice that is as variable as the institution performing the repair. While there are no robust data on the efficacy of any of these medical adjuncts for organ protection for the specific repair of DTAs or TAAAs, there is some non-human basic scientific evidence in its use for neurologic outcomes after circulatory arrest[13]. Following this, there have been some clinical studies which have suggested a trend towards improved neurologic outcomes with corticosteroid administration in patients undergoing surgical cases requiring DHCA[14]. However, in a retrospective study of 328 patients undergoing total arch replacement with moderate hypothermic arrest and selective antegrade cerebral perfusion, administration of large doses of methylprednisolone intraoperatively was not associated with any differences in neurologic outcomes[15]. In lieu of these conflicting data, there can be no formal recommendation of the administration of corticosteroids for the purpose of end-organ protection during DTA/TAAA repair using DHCA until the accumulation of more robust clinical evidence.
In addition to its immediate intraoperative and early post-operative negative sequelae as described previously, the main post-operative concern of DHCA utilization has been on the preservation of neurologic function following surgery. While there are documented movement disorders as well as reports of behavior and intellectual changes after both pediatric and adult cardiac surgery using DHCA, there is little in the literature specifically regarding DTA/TAAA repair. The aortic group at Yale published data on 394 patients undergoing thoracic aortic surgery using straight DHCA as their protection strategy, showing excellent clinical cognitive outcomes[16]. They went on to publish data on 29 patients from that cohort that were, by their definition, employed in careers requiring a high degree of cognitive ability, concluding that there were no perceptible or quantifiable changes in their cognitive capacity post-operatively, including memory function[17]. Overall, there is a robust published amount of data on the overall safety and tolerability of DHCA for various adult cardiac surgical procedures when used within acceptable margins of time, typically quoted at the most conservative to be around 30-35 min in the absence of adjunctive cerebral perfusion[18].
Technique
DHCA requires cannulation to facilitate cardiopulmonary bypass, including adequate venous drainage and arterial return. Cannulation is thus most often extra-mediastinal including femoral and/or jugular access for venous drainage and femoral, axillary, or distal aortic cannulation for arterial inflow, depending on aneurysmal extent. As CPB demands a high degree of systemic anticoagulation, most surgical dissection to facilitate repair is completed prior to cannulation to avoid unnecessary blood loss. Once surgical exposure is obtained or the limit to which dissection can be safely performed without cardiovascular support, heparin is systemically administered, and the patient is cannulated; when placing venous cannulas from the femoral vein, transesophageal echocardiography is often helpful in confirming adequate right atrial placement to provide maximum drainage[19]. Additional strategies to maximize venous drainage include concomitant jugular vein cannulation with a Y-connection, as well as utilization of vacuum-assisted venous drainage. Adequate venous output to the pump is a crucial consideration for aneurysm repair with CPB and DHCA, as inadequate drainage will yield suboptimal pump flows, leading to slower, incomplete systemic blood cooling, and once cardiac fibrillation occurs, inadequate cardiac drainage may precipitate distention as the heart can no longer eject.
A technical point worth further discussion is the specific conduct of peripheral CPB during the period of cooling and subsequent circulatory arrest. Flows equal or greater than 2 to 2.2 L/min/m2 are minimally sufficient to facilitate efficient cooling and subsequent rewarming[20]. Once CPB is established, cooling is initiated and generally requires at least 30 min[21]. During this period of cooling, the patient is fully heparinized and on cardiopulmonary bypass; the heart is emptied as much as possible by the venous cannula. During cooling and re-warming, the temperature gradient of blood coming from and returning to the patient during cooling is limited to 10 degrees Celsius to prevent generation of gaseous emboli. The duration of cooling should be at least thirty minutes or longer to minimize the effect of temperature alterations of tissue function[22,23].
Myocardial protection for DTA and TAAA repair is an issue present only with the use of deep hypothermia. As other perfusion strategies for DTA/TAAA repair utilize either LHB or partial CPB, both of which maintain spontaneous cardiac activity, there is no period of cardiac ischemia and thus no need for myocardial protection. In the process of achieving deep hypothermia for cerebral, spinal, and visceral protection, the heart will fibrillate, and coronary perfusion will become impaired, necessitating strategies for myocardial protection. The combination of hypothermia with induced cardiac arrest is a commonplace practice amongst contemporary cardiac surgeons. The greatest reduction in myocardial O2 consumption in the arrested heart occurs between 37 °C and 25 °C, with a relatively small decrease in energy requirements achieved thereafter[24]. Hypothermic fibrillatory arrest (HFA) will spontaneously occur, usually beyond temperatures of 28 to 30 degrees Celsius resulting in a reduced metabolic demand of the myocardium[25]. Major disadvantages of hypothermic fibrillatory arrest are the compromised subendocardial perfusion, particularly in patients with LV hypertrophy or LV distension particularly in patients in significant aortic regurgitation[24,26]. Ischemia, despite CPB-provided coronary perfusion, occurs due to the strength of the fibrillating myocardium exerting forces on subendocardial vasculature, as well as any potential intracavitary distention also exerting transmural pressure on these vessels. Both these situations are gravely exacerbated in the patient with left ventricular hypertrophy, in addition to their baseline elevated myocardial oxygen demand, placing this myocardium at elevated risk of ischemic complications[26]. Thus, patients with left ventricular hypertrophy are at higher risk of myocardial injury during the period of HFA because of subendocardial ischemia, despite ongoing continuous coronary perfusion provided by the pump. Cardiac distention is a universally present risk during all forms of extracorporeal circulatory support, including cardiopulmonary bypass, primarily due to the nature of retrograde flow towards the aortic valve with resultant higher afterload on the left ventricle, regardless of the cannulation strategy[27]. In patients with aortic insufficiency, the above situation is exacerbated by the incompetent valve yielding an elevated risk of cardiac chamber distention due to regurgitant flow from bypass. Other sources of blood can also contribute to left ventricular distention, including thebesian and bronchial vein flow, as well as incomplete cardiac drainage from the venous cannula[27]. All the above is present in the setting of a beating heart that can actively eject blood and thus decompress the left ventricle. However, to achieve deep hypothermic conditions to permit aortic surgery, the heart will experience spontaneous ventricular fibrillation, and all the above factors that may lead to left ventricular distention will thusly be further aggravated by a now fibrillating heart that can no longer eject, precipitating myocardial injury. There are numerous surgical strategies to decompress, or vent, the left ventricle and prevent myocardial injury because of distention: venting the left side of the heart directly, including pulmonary vein vent placement and left ventricular apical vent placement; additionally, the left heart can be vented indirectly from the right heart by using direct pulmonary artery vents, as well as percutaneously placed right sided vents into the pulmonary artery. While the potential deleterious effects of cardiac distention and non-uniform myocardial blood flow during hypothermic fibrillation are typically avoided by aortic cross-clamping and administration of cardioplegia, this typically requires proximal aortic access which is not afforded by the incisions used for conventional thoracoabdominal aortic surgery. Cardiac distention can be monitored for actively during surgery by using pulmonary arterial pressures, as well as transesophageal echocardiography, providing information on the necessity of left ventricular venting[28]. Cardiac distention can be exacerbated by incomplete venous drainage of the heart, particularly when femoral venous cannulation is employed. In the absence of direct visualization of the heart given the surgical exposure from the left chest, transesophageal echocardiography is crucial in ensuring adequate venous decompression of the right heart, which can be augmented by addition of vacuum assistance, larger cannulas, addition of other venous drainage cannulas, or cannula re-positioning. Additionally, some centers advocate administering a systemic dose of potassium chloride, from 40 to 60 mEq, into the CPB circuit to obtain diastolic arrest to maximize myocardial protection in addition to profound hypothermia[29].
Cerebral protection remains one of the critical objectives of safe DTA/TAAA repair, as these procedures continue to carry a 3%-8% perioperative stroke rate[30]. In assessing the points of the procedure for repair of DTA/TAAA that place the patient at risk of suffering neurologic complications, one can divide these into atheroembolism during the cooling and rewarming phase, and both atheroembolism and hypoperfusion during the proximal anastomotic phase. Patients with DTAs/TAAAs often have a high burden of atherosclerotic debris in their entire aorta, and the classic approach of placing patients on partial femoral cardiopulmonary bypass for cooling exposes the patient to the potential for retrograde atheroembolic debris propagation into their cerebral vasculature. With the use of femoral arterial cannulation, the establishment of cardiopulmonary bypass will initially be partial owing to both the incomplete decompression of the right heart, as well as ongoing left ventricle ejection and native cardiac output. During cooling, prior to hypothermic fibrillation, there will exist a duration of time with dual circulation, one being native antegrade cardiac output, the other being femoral arterial pump flow. Depending on the amount of drainage and subsequent femoral flow, as well as native cardiac output, there will be a varying location in the aorta where blood mixing will occur. As detailed in peripheral extracorporeal membrane oxygenation [ECMO] literature, the mixing point will typically be in the proximal aorta or aortic root at any ECMO flow rate in the presence of severe myocardial dysfunction, whereas this point will migrate more distally into the aortic arch as function improves[31]. It has been previously shown almost two decades ago that with normal myocardial performance, while the mixing point is beyond the aortic root even at maximal ECMO flows, it is typically within the aortic arch[32]. As such, it becomes clear that even at lower flows, there remains the possibility of the circulatory mixing point being in the aortic arch, raising the risk of retrograde embolism despite competing antegrade cardiac output. This could potentially be monitored with the use of right and left radial arterial lines, assessing for the loss of pulsatility in the left radial as a potential indicator that the left subclavian artery was no longer receiving inflow from native pulsatile flow, and that the mixing point had become more proximal. However, the combination of right axillary and femoral arterial access may both improve the safety and simplify the cooling and re-warming phases, as it would permit the use of antegrade axillary flow for cooling, reducing the risk of retrograde atheroembolism associated with retrograde femoral flow. Additionally, the ability to have retrograde femoral arterial flow may be beneficial both during DHCA for the proximal anastomosis with distal aortic clamping as well as the completion of visceral vessel incorporation, to maintain spinal cord collateral and lower body perfusion. Once all anastomoses are complete, re-warming can be still accomplished with the use of the antegrade axillary arterial line, once again avoiding the risk of retrograde embolization.
Regarding the performance of the proximal aortic anastomosis, both aortic clamp techniques and open aortic approaches with DHCA can increase the risk of neurologic complications from both embolism and hypoperfusion. While straight DHCA has been used with good outcomes in various cardiac surgical procedures, it is commonplace to supplement DHCA with additional methods of cerebral protection, classically in the form of antegrade or retrograde cerebral perfusion, to both increase the safe duration of circulatory arrest as well as potentially reduce post-operative neurologic complications. These techniques are easily implemented during cases involving standard median sternotomies, as it allows access to the base of the innominate artery for permitting antegrade cerebral perfusion from the right axillary artery, as well as access to the superior vena cava for retrograde cerebral perfusion. While it has been postulated that the risk of stroke associated with DTA/TAAA repairs is related to atheroembolic debris from arch clamping, large analyses between these types of cases treated with aortic cross-clamping or DHCA did not reveal a difference in post-operative stroke rates[8]. It can be inferred from these studies that the risk of stroke may be multifactorial, both from embolic causes in the case of arch clamping, as well as global hypoperfusion in relation to the use of DHCA. Thus, it remains an attractive option to potentially supplement DHCA with other adjunctive forms of cerebral protection conventionally used for central aortic repairs via sternotomy; however, there are anatomic challenges given the left thoracotomy exposure used for DTA/TAAA repairs. Regarding selective antegrade cerebral perfusion, there are multiple described techniques of delivering antegrade cerebral perfusion during DHCA from the left thoracotomy incision once the proximal aorta is opened via the insertion of balloon-tipped occlusion catheters into one or both innominate and left carotid arteries[33]. In this retrospective analysis of 30 patients to assess antegrade cerebral perfusion for DTA/TAAA repair, total circulatory arrest time independent of adjunctive cerebral perfusion remained the only identifiable risk factor for post-operative neurologic dysfunction, with no correlation identified between the use of antegrade cerebral perfusion and neurologic sequelae[33]. While maintaining cerebral perfusion may mitigate the risk of global hypoperfusion associated with DHCA, and while circulatory arrest may abrogate the risk of atheroembolism with aortic clamping, these described methods of achieving antegrade cerebral perfusion require both manipulation of the arch vessels with placement of catheters, as well as the potential for “sandblasting” effects of perfusion velocity jets from catheters into the cervical vessels, both of which may actually increase the risk of embolic phenomena. In lieu of this, it has been suggested that the use of retrograde cerebral perfusion may serve to reduce the risk of neurologic sequelae by flushing the cerebral vasculature of both air and embolic debris. Once again, retrograde cerebral perfusion is an established technique for surgical approaches via midline sternotomy with facile access to the superior vena cava, however it is more difficult to achieve this from the left thoracotomy position. In their retrospective analysis of 189 patients undergoing DTA/extent I/extent II TAAA repairs, Bavaria described their technique of combining retrograde cerebral perfusion with DHCA for cerebral protection. They either directly cannulated the inferior vena cava at the inferior cavoatrial junction from the left thoracotomy or inserted a percutaneous femoral venous cannula; in either situation, they ensured the tip was pointing towards the superior vena cava[34]. Using a connecting bridge between the arterial and venous cannulas, once DHCA was achieved and the aorta transected, they initiated flow from the arterial to venous cannula via the connecting bridge at a rate of 700-1,000 mL/min targeting a central venous pressure of 15 to 20 mmHg. Returned blood from the brachiocephalic arch vessels was collected via cardiotomy suctions to maintain retrograde flows and reservoir volume. They noted a 2.8% stroke rate with retrograde perfusion coupled with DHCA compared to a 12% stroke rate in the DHCA only cohort, despite the retrograde group having longer DHCA times. They point towards the ability of retrograde perfusion in flushing out air and debris from the cerebral vasculature as the likely mechanism of lower stroke rate. There have been other variations of retrograde flow in the repair of DTA/TAAAs in the literature, including distal aortic clamping and low-flow femoral arterial perfusion with steep Trendelenburg, allowing passive venous return to the right heart to fill the cerebral venous system and eventually drain out through the arch vessels. Nevertheless, the utilization of retrograde cerebral perfusion in the repair of DTA/TAAAs remains an attractive option for the potential reduction of embolic neurologic complications via the flushing of air and debris. In fact, one may combine these techniques by providing antegrade cerebral perfusion via selective carotid catheters during the proximal anastomotic creation, which can be removed, and retrograde cerebral perfusion instituted briefly to allow flushing of debris just prior to completion of the anastomosis, thereby combining the benefits of both methods, albeit at the expense of increased technical complexity. All these techniques will require further study prior to any recommendations being made on any of their superiority over others.
While there are numerous techniques described to improve the safety of DHCA in the repair of DTAs/TAAAs, it cannot go unstated that the development of newer hybrid surgical grafts will also improve the outcomes of these complex repairs. Many of the described adjunctive perfusion strategies aimed at reducing the risk of complications associated with DHCA suffer from both anatomic constraints and overall unfamiliarity of their execution in the context of a left thoracotomy incision. This has driven the development of newer hybrid grafts, such as the Terumo Thoraflex Plexus and Ante-Flo grafts, permitting intervention of DTAs and proximal thoracic aneurysms from a midline sternotomy, simplifying the surgical exposure as well as permitting the surgeon access to more familiar and facile techniques at cerebral protection[29]. Furthermore, these grafts can create a stable proximal landing zone for subsequent endovascular intervention of the thoracoabdominal aorta or provide a first stage repair that subsequently facilitates the second stage open thoracoabdominal repair. How these grafts are utilized and consequently compare in terms of post-operative outcomes and neurologic complications remains to be seen in reference to standard left thoracotomy approaches with various protection strategies.
DHCA provides acceptable cerebral protection. There is evidence in the literature to support aortic arch surgery for time periods of up to 40 min[35,36]. Other adjuncts which have been used to provide cerebral protection, either supplementally to deep hypothermia or with more milder temperatures, include antegrade and/or retrograde cerebral perfusion. Options for antegrade cerebral perfusion include right axillary cannulation, in addition to femoral cannulation for DTA and TAAA repair[37]. The benefits of using axillary artery perfusion in addition to femoral arterial perfusion are two-fold. Firstly, during cooling and fibrillation, cerebral blood flow is provided either partially or completely by retrograde femoral perfusion, which may pose an increased thromboembolic stroke risk, especially given the atheromatous nature of DTAs and TAAAs[38]. This risk is also manifested after completion of the proximal anastomosis and connection of the graft perfusion limb, as well as during re-warming and weaning off CPB, as until the heart resumes complete hemodynamic function with no venous drainage, a certain proportion of blood flow will remain retrograde. Secondly, during the proximal open anastomosis for DTAs/TAAAs during period of circulatory arrest, patients are placed in steep Trendelenburg position and various de-airing maneuvers are accomplished at the completion of the anastomosis to prevent cerebral air embolism. When re-perfusing the upper body with the perfusion limb of the aortic graft which is higher than the patient’s head, there is a risk of debris or air embolization that may be mitigated by re-perfusing from the axillary artery which would be lower than the graft and native aorta and thus better positioned to flush debris and air[38]. Thus, the left axillary artery can achieve similar results, although arguably still providing retrograde flow to the carotid vessels.
Corporal and spinal cord protection are also provided using deep hypothermia as a direct result of suppression of oxygen demand and metabolic requirements[39]. Several adjuncts can be using in combination with deep hypothermia for both visceral and spinal cord protection. Regarding the viscera, these include sequential clamping and maintaining perfusion with passive arterial shunting, LHB or CPB. If sequential clamping is not feasible, using individual catheters during LHB or CPB can perfuse target visceral vessels[40]. Regarding spinal cord protection, adjuncts include distal aortic perfusion in the form of LHB or CPB, utilizing sequential aortic clamping for immediate re-perfusion of reimplanted spinal arteries, cerebrospinal fluid drainage, central neurologic monitoring, and a host of potential pharmacologic agents[41].
As the brain is the most sensitive organ to ischemia, ensuring maximal attenuation of oxygen demand and metabolic rate with cooling is crucial prior to commencing circulatory arrest. There are a variety of methods to ensure the adequacy of hypothermia prior to the commencement of DHCA, ranging from allotting minimum periods of cooling time, various placements of temperature probes, the use of near-infrared spectroscopy [NIRS], electroencephalographic monitoring [EEG]. Bispectral index, the most common form of EEG utilization in aortic surgery, provides dual-channelled electroencephalographic data processed via a proprietary algorithm to provide a measure of cerebral metabolic activity, typically reaching a baseline of zero upon achievement of adequate DHCA[42]. The use of EEG to confirm adequate hypothermia is not strictly necessary, as it has been demonstrated that most patients achieve EEG quiescence after 45 min of cooling, correlating with a nasopharyngeal temperature of, on average,
ADVANTAGES
There are numerous advantages and disadvantages inherent in the use of DHCA for the repair of DTAs/TAAAs, which will be both delineated below, as well as listed in Table 1.
Advantages and disadvantages of deep hypothermic circulatory arrest in the repair of thoracoabdominal aortic aneurysms
Advantages | Disadvantages | |
Deep hypothermic circulatory arrest | Non-clampable distal aortic arch or proximal descending thoracic aorta due to large aneurysms, distorted anatomy, or need for fenestration in aortic dissection Additional spinal and visceral protection associated with hypothermia | Full heparization for cardiopulmonary bypass Coagulopathy associated with deep hypothermia Difficult to provide cerebral perfusion during circulatory arrest Potential for cardiac ischemia during ventricular fibrillation associated with hypothermia Pulmonary injury associated with coagulopathy, pulmonary edema, and cardiopulmonary bypass |
Utilization of CPB with brief periods of DHCA for the repair of DTAAs and TAAAs has numerous advantages compared to other approaches, including “clamp-and-sew,” left heart bypass, and partial cardiopulmonary bypass. The ability to operate in a bloodless surgical field can make crucial differences in the expeditious performance of the procedure, particularly during more challenging moments such as the creation of the proximal anastomosis[44]. The elimination of the need for proximal aortic clamping is one of the primary advantages of DHCA, which has multiple included benefits. Firstly, the amount of healthy aortic tissue used for construction of a proximal anastomosis is often compromised by the requirement of a cross-clamp, which may unnecessarily lead to changing the location of the anastomosis to a more proximal location with more available tissue and increase surgical complexity and risk. Secondly, even after identification of a suitable proximal anastomotic site and associated clamp site, more extensive periaortic dissection is required for placement of a clamp than is necessary when using DHCA, which may increase risk of bleeding, injury to the recurrent laryngeal or phrenic nerve, as well as increased aortic manipulation which may lead to atheroembolic events[8]. Lastly, the unparalleled endoluminal exposure of the proximal aortic anastomosis site during DHCA allows for inspection of the quality of aortic tissue and optimal suture placement, as well as debridement of intra-aortic debris which may serve to reduce the incidence of thromboembolism[19].
An additional advantage of utilizing DHCA relates to the ability of having an open proximal aorta. During the creation of the proximal anastomosis, ongoing low-flow lower body perfusion with partial occlusion of venous drainage of the right heart leads to retrograde flow into the superior vena cava, resulting in gentle flushing of the innominate and left carotid arteries, removing air and debris[19]. Increasing lower body flows and volume loading the patient can also be performed as well just prior to graft clamping and restoration of circulation to flush the cerebral vasculature and proximal aorta to clear debris[19].
Regardless of the various strategies used to facilitate DTAA/TAAA repair, there is a necessary reduction of blood flow to the spinal cord [the degree to which varies depending on the extent of the aneurysm, previous surgical intervention, and adjunctive perfusion strategies] as well as periods of absolute ischemia to end-organs requiring target vessel anastomosis to the aortic graft [renal and visceral vessels]. DHCA provides an effective method to off-set the substantial risks of spinal cord and renovisceral ischemia during these operations. Kouchoukos has published multiple results on the routine use of DHCA for the repair of DTAs and TAAAs, with particular focus on spinal cord and visceral protection, noting adequate protection during periods of circulatory arrest without the need for other adjuncts[16]. Spinal cord ischemia and subsequent postoperative paraplegia is arguably the most devastating complication following open repair of DTAs/TAAAs. Based on multiple laboratory-based models and clinical imaging studies, spinal cord injury following DTA/TAAA repair followed the pathophysiology of an ischemic-reperfusion model, wherein temporary aortic cross-clamping induces hypoperfusion to the distal spinal cord vessels, and re-perfusion further exacerbates this insult[45]. Systemic hypothermia is one of the most reliable adjuncts for the prevention of spinal cord ischemia as well as mitigating the deleterious effects of reperfusion and is thus used by many surgeons during complex aortic intervention, providing uniform spinal cord cooling[4]. When DHCA is not used, other surgical adjuncts are frequently employed to accomplish similar reductions in spinal ischemic time, such as epidural spinal cord cooling, perfusion catheters, partial CPB, or left heart bypass [LHB]. Left heart bypass is an excellent technique at proximal aortic decompression and distal aortic perfusion to maintain blood flow to vessels beyond the distal aortic cross-clamp, including intercostal, lumbar, and hypogastric vessels, providing ongoing in-line flow to the spinal cord during occlusion of other major inflow sources. It is widely reported that distal aortic perfusion pressure must be equal to or greater than 60mmHg to minimize spinal cord injury, and this is readily accomplished with centrifugal pumps used in LHB[46]. However, when using LHB, one must accept the lack of venous reservoir, ability to efficiently cool and rewarm the patient, and rely on intrinsic lung function for gas exchange which may be impaired due to the necessity of single lung ventilation. Furthermore, if the extent of the aneurysm requires aortic arch clamping proximal to the left subclavian artery for proximal anastomosis creation, this temporary occlusion will affect anterior spinal cord artery perfusion, potentially compromising spinal cord blood flow[25]. To overcome these deficiencies, at the expense of higher doses of systemic heparinization, partial CPB can be utilized, allowing for optimal control of oxygenation; this can be converted to full CPB for cerebral and myocardial perfusion with well-oxygenated blood when there is additional right axillary cannulation, and if necessary, cool the patient for a period of DHCA. In summary, utilization of DHCA certainly offers a high degree of protection to the spinal cord simply by virtue of direct reduction of metabolic demands. Its use removes the uncertainty and technical complexity associated with relying on other protective strategies such as selective segmental artery perfusion with various balloon-tipped catheter techniques. Assessment of the aorta during periods of DHCA minimizes the duration of time with back-bleeding spinal arteries, which may reduce spinal cord steal phenomena[18]. However, ligation of intercostal or lumbar arteries prior to or after aneurysm sac opening can also be equally performed. Lastly, the use of DHCA for the proximal anastomosis can be augmented, rather than replaced, by similar adjunctive protection strategies for DTA/TAAA repair that rely on the use of a proximal cross-clamp and distal perfusion. While the practice of sequential clamping with distal perfusion is mostly utilized with LHB, one can equally cool the patient and utilize DHCA for an open proximal anastomosis, taking advantage of the hypothermic protection on the spinal cord, as well as utilize a distal aortic clamp with lower femoral flow rates to augment hypothermic flow to the spinal cord in a manner analogous to antegrade cerebral perfusion during DHCA for arch repair.
Analogously, DHCA simplifies the management of renovisceral artery protection. DHCA alone provides simple yet robust protection of the kidneys and abdominal viscera, however the operative time required to perform the proximal anastomosis, along with spinal reimplantation, and subsequently incorporate the renovisceral vessel anastomoses as either separate bypasses or a patch into the aortic graft substantially increases the DHCA time, with its inherent risks. The benefits of DHCA on renovisceral protection essentially lie within the degree of protection it affords during creation of the proximal anastomosis. In this regard, DHCA has a more protective effect than other perfusion techniques particularly when organ ischemic time approaches or exceeds 60 min[5]. Once there is no longer a need for circulatory arrest, resumption of circulation can be combined with the achieved level of deep hypothermia to provide ongoing visceral protection while resuming perfusion to the brain and/or lower body, depending on cannulation techniques.
DISADVANTAGES
Utilization of both CPB and periods of DHCA are necessarily accompanied by a much greater degree of systemic heparinization, exposure to the cardiopulmonary bypass circuit, and deleterious physiologic effects systemic hypothermia compared to many of the other modalities of organ protection for DTA and TAAA repair, representing a distinct inherent disadvantage. The degree of hypothermia has known sequelae, including bleeding and coagulopathy, exacerbated by the degree of heparinization needed for CPB and the duration of surgery required to achieve thermic extremes[25]. Pulmonary injury can occur due to both cold temperatures and retraction to the heparinized lung during DHCA, and the systemic inflammatory response of cardiopulmonary bypass is exageerated with DHCA, as the blood exposure to the bypass circuit is necessarily increased as a function of the time required for cooling and subsequent re-warming[9]. Inherent in this disadvantage is the increased operative times due to cooling and re-warming. Air and coronary artery embolism are risks associated with DHCA due to an open aorta without cross-clamp protection[12].
As DHCA requires CPB, there are risks manifest to the selected method of placing the patient on pump and the resultant necessity of managing hemodynamics with extracorporeal support during while on CPB. As DTA and TAAA repair are performed via left thoracotomies, when circulatory support is desired, this is most often accomplished with femoral cannulation and resultant retrograde arterial flow. With femoral cannulation, there are substantive risks of retrograde embolization of aortic air and debris, exacerbated by the increased amount of time required to use retrograde perfusion both during cooling and re-warming[8]. When femoral cannulation is used in combination with DHCA, however, one can take advantage of the open proximal aortic near the end of the completion of the proximal anastomosis by infusing the venous line to flush debris from the graft. Once the necessity for circulatory arrest is complete, typically upon creation of the proximal anastomosis, most chosen grafts will have a perfusion side-arm which permits application of a clamp on the graft just distal to both the proximal anastomosis and perfusion arm, allowing for resumption of perfusion to the upper body and brain. Alternatively, arterial in-flow for CPB for these cases may be axillary artery cannulation, eliminating the potential risks associated with retrograde aortic flow. Central cannulation with access to the ascending aorta from a left thoracotomy can also accomplish these goals but may be limited in certain patients who have had previous cardiothoracic surgery or have challenging anatomy. Additional techniques may employ femoral arterial or distal aortic cannulation to allow for perfusion to the lower body and spinal cord with the use of a distal aortic cross clamp for both low-flow perfusion as well as the afforded protection of deep hypothermia.
Myocardial protection is an important consideration when using DHCA to facilitate DTAA or TAAA repair. As described earlier in this paper, systemic hypothermia can serve to protect the myocardium during circulatory arrest, however this may come at the expense of exposing to many points of potential injury. During the process of cooling, the heart will experience perfused hypothermic fibrillation and subsequent fibrillatory arrest and during re-waring there is typically spontaneous defibrillation around
Additional risks include the potential for cerebral edema and ischemia, as well as pulmonary edema. As temperature drops, there is loss of cerebral autoregulation, and cerebral blood flow becomes dependent on mean arterial pressure, increasing the risk of cerebral edema and increased cerebrovascular exposure to potential emboli[47]. Cerebral ischemia is a multifactorial consequence of DHCA, purportedly due to excessive metabolic demand in the form of oxygen debt, microvascular no-reflow phenomena, and derangements to cerebral autoregulation[48,49]. These alterations can persist well beyond the immediate post-operative period and can also stochastically occur hours to days after the index operation even if immediate absent. While there are qualitative concerns regarding neurocognitive performance after DHCA, more recently published quantitative data suggests there is no difference compared to non-DHCA patients undergoing cardiac surgery. 48 Pulmonary edema is a known consequence of CPB utilization due to ischemia-reperfusion injury; this is exacerbated with the use of DHCA due to duration of CPB, as well as temperature injury along with the risks of pulmonary contusion[23,50]. The incidence of tracheostomy is up to 9% in patients undergoing TAAA repair with DHCA[39].
OUTCOMES
The effectiveness of DHCA as a strategy of organ protection to facilitate complex aortic reconstruction is primarily because of temperature reduction of decreasing organ metabolic rate, shielding the tissue from the detrimental effect of ischemia. Most surgical strategies using DHCA include reperfusion of the brain and upper body after proximal anastomosis by using either axillary artery cannulation or a side branch of the newly placed aortic graft and placing a clamp distally on the graft. However, there is variable techniques described in the literature as to whether re-warming is initiated at this point, or deep hypothermia is maintained for distal organ protection despite cross-clamp and resumption of circulation to the upper body. If deep hypothermia is continued, so too are the inherent risks associated. The benefits of avoiding proximal and/or sequential aortic cross-clamping, with the inherent need to mobilize and dissect around the aorta, as well as maintenance of a bloodless field are well-described and can also be extrapolated from the data of DHCA use with proximal aortic repairs[49]. Despite the robust literature on the safety and efficacy of DHCA for aortic surgery, there are numerous proponents of alternate techniques that involve proximal aortic cross-clamping and distal perfusion, with or without adjuncts including sequential aortic clamping, milder hypothermia, cerebrospinal fluid drainage, epidural cooling, intrathecal papaverine, and cold renovisceral vessel perfusate, amongst others[49]. Conceptually, these techniques avoid the risks of profound hypothermia as well as circulatory arrest. These include partial cardiopulmonary bypass using femoral venous cannulation as well as left-heart bypass, both of which are performed with a beating heart and the presence of a proximal cross-clamp. The efficacy and outcomes of DTA and TAAA repair using DHCA must be evaluated both in isolation as well as in reference to these alternative repair strategies. Kouchoukos et al. present ed their 30-year experience using DHCA on TAAA repair, analyzing early 30-day outcomes on 285 patients, publishing their rates of post-operative stroke, spinal cord injury, and permanent end-organ dysfunction, amongst the lowest in the literature for open surgery on the thoracoabdominal aorta[39]. A meta-analysis of nearly 10,000 patients undergoing open TAAA repair using various techniques to mitigate organ system injury demonstrated an early 30-day mortality of 11.26%, comparable to the published results from Kouchoukos of 7.4%[3]. When directly assessing the outcomes of DTA/TAAA repair between DHCA and non-DHCA techniques, it is important to consider the inherent limitations of comparing the two. First and foremost, there have been no randomized clinical studies prospectively assessing the outcomes of these two techniques on comparable patient populations. Most studies are limited to retrospective single-centre reported outcomes, and the meta-analyses that coalesce their outcomes. The decision to pursue one technique over another is often due to differing institutional approaches to certain anatomic factors or disease extent, as well as surgeon preference, co-morbid patient factors, and potentially the emergent nature of the repair. Weiss et al. conducted a propensity score-matched comparison of deep versus mild hypothermia for TAAA repair[25]. They assessed 90 patients for reversible and permanent outcomes, including renal and liver failure, paraplegia and 30-day mortality, finding an improved postoperative outcome rates for reversible adverse outcomes compared to non-DHCA techniques[25]. In contrast,
SUMMARY
DHCA to facilitate DTA/TAAA repair is an established technique with favorable outcomes. It obviates the need for proximal cross clamping of hostile distal aortic arches or proximal descending aneurysms; need for fenestration of the aortic arch; and improved organ protection by reducing oxygen consumption. However, this is countered by the potential adverse effects on the myocardial, cerebral, and pulmonary systems, along with the increased coagulopathy. The use of DHCA remains a clinical decision guided by the experience and preferences of the surgical team as well as the technical demands of the proposed surgery.
DECLARATIONS
Authors’ contributionsContributed in the design, literature review, and writing of the manuscript: Troncone MJ, Hong JC
Availability of data and materialsNot applicable.
Financial support and sponsorshipNone.
Conflicts of interestAll authors declared that there are no conflicts of interest.
Ethical approval and consent to participateNot applicable.
Consent for publicationNot applicable.
Copyright© The Author(s) 2023.
REFERENCES
1. Coselli J, Lemaire S, Preventza O. Outcomes of 3309 thoracoabdominal aortic aneurysm repairs. J Vasc Surg 2016;151:1323-37.
2. MacArthur RG, Carter SA, Coselli JS, LeMaire SA. Organ protection during thoracoabdominal aortic surgery: rationale for a multimodality approach. Semin Cardiothorac Vasc Anesth 2005;9:143-9.
3. Moulakakis KG, Karaolanis G, Antonopoulos CN, et al. Open repair of thoracoabdominal aortic aneurysms in experienced centers. J Vasc Surg 2018;68:634-645.e12.
4. Waked K, Schepens M. State-of the-art review on the renal and visceral protection during open thoracoabdominal aortic aneurysm repair. J Vis Surg 2018;4:31.
5. Nathan DP, Brinster CJ, Woo EY, Carpenter JP, Fairman RM, Jackson BM. Predictors of early and late mortality following open extent IV thoracoabdominal aortic aneurysm repair in a large contemporary single-center experience. J Vasc Surg 2011;53:299-306.
6. Gaudino M, Khan FM, Rahouma M, et al. Spinal cord injury after open and endovascular repair of descending thoracic and thoracoabdominal aortic aneurysms: a meta-analysis. J Thorac Cardiovasc Surg 2022;163:552-64.
7. Gilling-Smith GL, Worswick L, Knight PF, Wolfe JH, Mansfield AO. Surgical repair of thoracoabdominal aortic aneurysm: 10 years' experience. Br J Surg 1995;82:624-9.
8. Yoo JS, Kim JB, Joo Y, et al. Deep hypothermic circulatory arrest versus non-deep hypothermic beating heart strategy in descending thoracic or thoracoabdominal aortic surgery. Eur J Cardiothorac Surg 2014;46:678-84.
9. Girardi LN, Krieger KH, Mack CA, Lee LY, Tortolani AJ, Isom OW. Does cross-clamping the arch increase the risk of descending thoracic and thoracoabdominal aneurysm repair? Ann Thorac Surg 2005;79:133-7; discussion 137.
10. Hamilton C, Engelhardt B, Weinbrenner F, et al. Literature review of large patients undergoing cardio pulmonary bypass: concerns, management and future considerations. KARDIOTECHNIK 2020:124.
11. Molnar J, Colah S, Larobina M, Large SR, Arrowsmith JE, Klein AA. Cardiopulmonary bypass and deep hypothermic circulatory arrest in a massively obese patient. Perfusion 2008;23:243-5.
13. Shum-Tim D, Tchervenkov CI, Jamal AM, et al. Systemic steroid pretreatment improves cerebral protection after circulatory arrest. Ann Thorac Surg 2001;72:1465-71; discussion 1471.
14. Krüger T, Hoffmann I, Blettner M, Borger MA, Schlensak C, Weigang E. GERAADA Investigators. Intraoperative neuroprotective drugs without beneficial effects? Eur J Cardiothorac Surg 2013;44:939-46.
15. Yan S, Gao S, Lou S, et al. Does methylprednisolone provide protective effect in total aortic arch replacement requiring hypothermia circulatory arrest and selective cerebral perfusion? Perfusion ;2022:2676591221113650.
16. Gega A, Rizzo JA, Johnson MH, Tranquilli M, Farkas EA, Elefteriades JA. Straight deep hypothermic arrest: experience in 394 patients supports its effectiveness as a sole means of brain preservation. Ann Thorac Surg 2007;84:759-66; discussion 766.
17. Percy A, Widman S, Rizzo JA, Tranquilli M, Elefteriades JA. Deep hypothermic circulatory arrest in patients with high cognitive needs: full preservation of cognitive abilities. Ann Thorac Surg 2009;87:117-23.
18. Gravlee G P, Davis R F, Hammon J, et al. Cardiopulmonary bypass and mechanical support: principles and practice. 4th ed. Philadelphia: Lippincott Williams & Wilkins; 2015.
19. Coselli JS, Bozinovski J, Cheung C. Hypothermic circulatory arrest: safety and efficacy in the operative treatment of descending and thoracoabdominal aortic aneurysms. Ann Thorac Surg 2008;85:956-63; discussion 964.
20. Kouchoukos NT, Wareing TH, Izumoto H, Klausing W, Abboud N. Elective hypothermic cardiopulmonary bypass and circulatory arrest for spinal cord protection during operations on the thoracoabdominal aorta. J Thorac Cardiovasc Surg 1990;99:659-64.
21. Harrington DK, Fragomeni F, Bonser RS. Cerebral perfusion. Ann Thorac Surg 2007;83:S799-804; discussion S824.
22. Gocoł R, Hudziak D, Bis J, et al. The role of deep hypothermia in cardiac surgery. Int J Environ Res Public Health 2021;18:7061.
23. Engelman R, Baker RA, Likosky DS, et al. The society of thoracic surgeons, the society of cardiovascular anesthesiologists, and the American society of extracorporeal technology: clinical practice guidelines for cardiopulmonary bypas-temperature management during cardiopulmonary bypass. J Extra Corpor Technol 2015;47:145-154.
24. Buckberg GD, Hottenrott CE. Ventricular fibrillation. Its effect on myocardial flow, distribution, and performance. Ann Thorac Surg 1975;20:76-85.
25. Weiss AJ, Lin HM, Bischoff MS, et al. A propensity score-matched comparison of deep versus mild hypothermia during thoracoabdominal aortic surgery. J Thorac Cardiovasc Surg 2012;143:186-93.
26. Elghobary T, Ali IM, Ahmad AF. Operative myocardial protection in patients with left ventricular hypertrophy: the role of systemic hypothermia. Open J Cardiovasc Surg 2011;4:1-4.
27. Cevasco M, Takayama H, Ando M, Garan AR, Naka Y, Takeda K. Left ventricular distension and venting strategies for patients on venoarterial extracorporeal membrane oxygenation. J Thorac Dis 2019;11:1676-83.
28. Crawford ES, Coselli JS, Safi HJ. Partial cardiopulmonary bypass, hypothermic circulatory arrest, and posterolateral exposure for thoracic aortic aneurysm operation. J Thorac Cardiovasc Surg 1987;94:824-7.
29. Fehrenbacher JW, Hart DW, Huddleston E, Siderys H, Rice C. Optimal end-organ protection for thoracic and thoracoabdominal aortic aneurysm repair using deep hypothermic circulatory arrest. Ann Thorac Surg 2007;83:1041-6.
30. Piazza M, Ricotta JJ 2nd. Open surgical repair of thoracoabdominal aortic aneurysms. Ann Vasc Surg 2012;26:600-5.
31. Annich GM, Lynch WR, MacLaren G, Wilson JM, Bartlett RH. ECMO: extracorporeal cardiopulmonary support in critical care. Washington: University of Washington Press; 2018.
32. Kato J, Seo T, Ando H, Takagi H, Ito T. Coronary arterial perfusion during venoarterial extracorporeal membrane oxygenation. J Thorac Cardiovasc Surg 1996;111:630-6.
33. Hosoyama K, Kawamoto S, Kumagai K, et al. Selective cerebral perfusion with the open proximal technique during descending thoracic or thoracoabdominal aortic repair: an option of choice to reduce neurologic complications. Ann Thorac Cardiovasc Surg 2018;24:89-96.
34. Kawajiri H, Saran N, Dearani JA, et al. Whole body retrograde perfusion combined with central aortic perfusion strategy in the repair of distal arch pathology through a lateral thoracotomy. Mayo Clin Proc 2023;98:432-42.
35. Ziganshin BA, Rajbanshi BG, Tranquilli M, Fang H, Rizzo JA, Elefteriades JA. Straight deep hypothermic circulatory arrest for cerebral protection during aortic arch surgery: Safe and effective. J Thorac Cardiovasc Surg 2014;148:888-98; discussion 898.
36. Damberg A, Carino D, Charilaou P, et al. Favorable late survival after aortic surgery under straight deep hypothermic circulatory arrest. J Thorac Cardiovasc Surg 2017;154:1831-1839.e1.
37. Kawaharada N, Ito T, Naraoka S, et al. Right axillary arterial perfusion for descending thoracic or thoracoabdominal aortic aneurysm repair with open proximal anastomosis through left thoracotomy. Gen Thorac Cardiovasc Surg 2014;62:547-52.
38. Sabik JF, Lytle BW, McCarthy PM, Cosgrove DM. Axillary artery: an alternative site of arterial cannulation for patients with extensive aortic and peripheral vascular disease. J Thorac Cardiovasc Surg 1995;109:885-90; discussion 890.
39. Kouchoukos NT, Kulik A, Haynes M, Castner CF. Early outcomes after thoracoabdominal aortic aneurysm repair with hypothermic circulatory arrest. Ann Thorac Surg 2019;108:1338-43.
40. Aftab M, Coselli JS. Renal and visceral protection in thoracoabdominal aortic surgery. J Thorac Cardiovasc Surg 2014;148:2963-6.
41. Kemp CM, Feng Z, Aftab M, Reece TB. Preventing spinal cord injury following thoracoabdominal aortic aneurysm repair: The battle to eliminate paraplegia. JTCVS Tech 2021;8:11-5.
42. Falasa MP, Arnaoutakis GJ, Janelle GM, Beaver TM. Neuromonitoring and neuroprotection advances for aortic arch surgery. JTCVS Tech 2021;7:11-9.
43. Bavaria JE, Pochettino A, Brinster DR, et al. New paradigms and improved results for the surgical treatment of acute type A dissection. Ann Surg 2001;234:336-42; discussion 342.
44. Kouchoukos NT. Thoracoabdominal aortic aneurysm repair using hypothermic cardiopulmonary bypass and circulatory arrest. Ann Cardiothorac Surg 2012;1:409-11.
45. Kawaharada N, Ito T, Koyanagi T, et al. Spinal cord protection with selective spinal perfusion during descending thoracic and thoracoabdominal aortic surgery. Interact Cardiovasc Thorac Surg 2010;10:986-90; discussion 990.
46. Wan IY, Angelini GD, Bryan AJ, Ryder I, Underwood MJ. Prevention of spinal cord ischaemia during descending thoracic and thoracoabdominal aortic surgery. Eur J Cardiothorac Surg 2001;19:203-13.
47. Miyamoto TA, Miyamoto KJ. Is alpha-stat management still justified for deep hypothermic circulatory arrest in adults? J Thorac Cardiovasc Surg 1999;118:569-70.
48. Chau KH, Friedman T, Tranquilli M, Elefteriades JA. Deep hypothermic circulatory arrest effectively preserves neurocognitive function. Ann Thorac Surg 2013;96:1553-9.
49. Alhussaini M, Falasa MP, Jeng EI, et al. Application of deep hypothermic circulatory arrest in open left chest aortic aneurysm repair. J Thorac Cardiovasc Surg ;2021:S0022-5223.
Cite This Article
Export citation file: BibTeX | RIS
OAE Style
Troncone MJ, Hong JC. Descending thoracic and thoracoabdominal aortic aneurysm repair using deep hypothermic circulatory arrest. Vessel Plus 2023;7:14. http://dx.doi.org/10.20517/2574-1209.2023.08
AMA Style
Troncone MJ, Hong JC. Descending thoracic and thoracoabdominal aortic aneurysm repair using deep hypothermic circulatory arrest. Vessel Plus. 2023; 7: 14. http://dx.doi.org/10.20517/2574-1209.2023.08
Chicago/Turabian Style
Troncone, Michael J., Jonathan C. Hong. 2023. "Descending thoracic and thoracoabdominal aortic aneurysm repair using deep hypothermic circulatory arrest" Vessel Plus. 7: 14. http://dx.doi.org/10.20517/2574-1209.2023.08
ACS Style
Troncone, MJ.; Hong JC. Descending thoracic and thoracoabdominal aortic aneurysm repair using deep hypothermic circulatory arrest. Vessel Plus. 2023, 7, 14. http://dx.doi.org/10.20517/2574-1209.2023.08
About This Article
Special Issue
Copyright
Data & Comments
Data
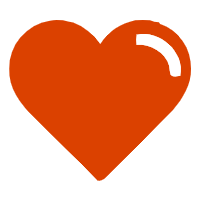

Comments
Comments must be written in English. Spam, offensive content, impersonation, and private information will not be permitted. If any comment is reported and identified as inappropriate content by OAE staff, the comment will be removed without notice. If you have any queries or need any help, please contact us at support@oaepublish.com.